Present and future perspectives on total artificial hearts
Introduction: the pioneer trail
Total replacement of the failing heart with a mechanical pump has been the Holy Grail for cardiac surgeons for decades. The early years of heart transplantation and development of an artificial heart represent a fascinating and controversial part of the history of medicine in the last century. Moving forward with artificial cardiac replacement required physicians to break away from the ethical conventions established by others. Initial efforts at creating a complete cardiac replacement artificial organ began as one of the several scientific initiatives during the Kennedy administration in the 1960s. William Kolff and Michael DeBakey were among the pioneers of artificial heart development. During these years, the National Institutes of Health (NIH) were a very important source of funding with grants and contracts for scientists and engineers, becoming a catalyst for artificial heart research. There were at least four academic institutions in the USA with robust research programs working on developing artificial hearts for clinical applications: William Kolff’s team at the University of Utah, Michael Debakey’s team at the Baylor College of Medicine, William Pierce’s team at Pennsylvania State University, and Yuki Nosé’s team at the Cleveland Clinic. There were also artificial heart research programs underway in academic centers in Japan, West Germany, East Germany, Czechoslovakia and the Soviet Union. Researchers were working on improving device designs and materials, performance, durability and outcome measures through extensive bench research and animal experiments.
The first human implant took place in 1969 at the Texas Heart Institute in Houston. Denton Cooley implanted a pneumatic Liotta total artificial heart (TAH) as a BTT in 47-year-old patient who could not be weaned from cardiopulmonary bypass. After 64 hours of support with the TAH, the patient underwent cardiac transplantation but eventually died of organ rejection 32 hours later. Many of the scientists at the time thought this operation was premature, but it paved the way for the next clinical era of TAHs. The next human implant took place in 1981. The operation was again performed in Houston, Texas by Dr. Cooley as a BTT. He waited 12 years before re-attempting the implantation of a TAH. This time, Cooley implanted a pneumatic Akutsu TAH. This device was made of an improved synthetic material, polyurethane, but it still inflated by compressed air. The device provided 39 hours of support for the patient, who died shortly after his transplant surgery.
The third human implant was performed in 1982 at the University of Utah by Dr. William DeVries in a 61-year-old patient, Barney Clark. This was the first time that a TAH was implanted as a permanent replacement for a natural heart. The Jarvik 7 TAH, developed in Kolff’s lab, was named after Dr. Robert Jarvik, the most recent designer of the device. Clark never left the hospital, as he was tethered to the air compressor with a size of a washing machine, which powered his artificial heart. He experienced various adverse events and eventually died after 112 days of support. Three more patients in the USA and one patient in Sweden were implanted with the Jarvik 7 as a permanent replacement device in 1984 and 1985. The longest survivor was William Schroeder, who lived for 620 days.
Despite the great enthusiasm during the pioneering years, the goal to complete cardiac replacement was too extensive for the level of scientific, clinical and social maturation of the era, and many scientists believed that this was not the technology of the future.
The National Heart Institute decided to end its funding of the technology. The research in the field of MCS was focused on VADs, where remarkable advancements in reliability and miniaturization of devices were achieved in just two decades.
Many research projects on TAH at sites such as Phoenix, Penn State, Berlin, Vienna, Brno, Unger, Poisk-IOM, were stopped after a few clinical implants, mainly due to complications which limited the lifespan of early human recipients to days.
Nevertheless, after the first five successful cases, the Jarvik 7 heart became more widely used as a temporary TAH bridging patients to transplant. Since 1982, more than 1,300 patients have used the Jarvik 7 heart. The company’s ownership has changed hands several times and it was eventually renamed as the CardioWest heart. After a 10-year long clinical study involving 95 patients, this TAH produced a 79% success rate for BTT with excellent overall survival, including post-transplantation (1). In 2004, the FDA granted their approval, making CardioWest the first and still the only fully approved TAH in USA. In 2006, it was CE marked as well.
From the pioneering era to date, only one implantable replacement heart, the AbioCor TAH, received FDA approval in 2006, under the Humanitarian Use Device (HUD) provision for DT. At present, another implantable TAH, the Carmat TAH, has just started a clinical trial in France.
The present: do we really need TAHs?
Between June 23, 2006, and December 31, 2013, 10,542 patients who received an FDA approved durable MCS device were entered in the Interagency Registry for Mechanically Assisted Circulatory Support (INTERMACS) database (2). The rate of implants per year has increased from 338 devices implanted a year in 2007 (first full year of data collection) to 2506 in 2013.
The dominance of continuous-flow left ventricular assist device (LVAD) technology has emerged since the approval of the HeartMate II device (Thoratec, Pleasanton, California, USA) in 2008 for BTT therapy and for long-term DT in 2010. In 2013, more than 42% of implants had been designated as DT. In contrast, the implant rate of the only FDA approved TAH, the Syncardia CardioWest, has moved from 22 devices implanted a year in 2007 to 66 a year in 2013 according to the INTERMACS registry. One might conclude that even in the context of biventricular failure, the improvement in flow through the systemic circulation achieved by use of an LVAD is sufficient to achieve a renal improvement in hemodynamic performance and organ function for many patients. However, INTERMACS reported that right heart failure is the primary cause of death in 4.7% of patients, while 2.8% of patients die of intractable arrhythmias and 3.4% of congestive heart failure during MCS, identifying a group of patients that might benefit from biventricular support. Moreover, besides overt biventricular failure, other cardiac pathologies that might contraindicate LVAD implantation include infiltrative cardiomyopathies (amyloid), restrictive cardiomyopathies, cardiac malignancies, post infarction VSD and the presence of heart valve mechanical prostheses. If the patient’s condition does not allow concomitant heart surgery at the time of LVAD implantation to correct aortic regurgitation, cardiac aneurysm, or congenital heart malfunction, TAH implantation might be preferred. Finally, according to the International Society of Heart Lung Transplantation (3), cardiac graft failure is the major cause of death among heart-transplant patients, accounting for 44% of transplant deaths within five years. Despite this, only 2% of patients with a failed transplant can get a new heart transplant, largely due to the shortage of donor hearts. The TAH may be a superior therapy for such patients (4). Moreover, the fourth INTERMACS report showed improved survival at three and six months among patients with biventricular dysfunction supported with a TAH versus a BiVAD (5). The recent report from Groupe de Relexion sur l’Assistance Mecanique (GRAM) registry showed that Syncardia recipients experienced significantly fewer neurologic events compared to BiVAD recipients. There are likely design elements of the Syncardia which may be responsible for reduced thromboembolic events (6). These data supports the adoption of TAH over BiVAD for biventricular support patients with INTERMACS class I and II.
Syncardia INC has reported an increase in support time for patients worldwide. This can be attributed to the paucity of organ donors and the increasing expertise achieved in managing patients with a TAH. More than 92 patients have been supported with a CardioWest TAH for more than one year (Table 1). Interestingly, considering the growing adoption of LVAD as DT underlined by INTERMACS registry VI, the use of TAH as long-term support has been recently investigated in 47 patients supported for more than one year with a CardioWest TAH (7). The rate of successful transplantation in this cohort of patients was greater than 70%. Only two patients of 27 died due to device related problems (membrane rupture), and infections still represent the major limit of this bulky device. The long-term support record is held by an Italian patient, who was supported for 1,373 days with a CardioWest TAH before a successful transplant.
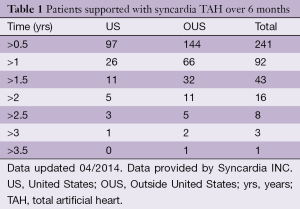
Full table
Considering the therapeutic need for TAHs, there really is a necessity for this device. The scarce adoption of TAHs for clinical use depends mostly on the technology limitations inherent to the TAHs currently available.
TAHs: state of the art
CardioWest TAH (SynCardia, Tucson, Arizona, USA) has a very simple design. It delivers pulsatile flow by filling two artificial ventricles lined with polyurethane, and ejects blood via a four-layer, pneumatically driven, moving diaphragm (8). Four mechanical valves (formerly Medtronic Hall, recently proprietary Sin HallTM, with identical design, materials and manufacturing processes) guarantee unidirectional flow. The ventricles are connected to patient’s circulatory system by cuffs sutured to the atria and vascular grafts sutured to the aorta and pulmonary artery. At maximal stroke volume (70 mL), it delivers a cardiac output of more than 9 L per minute.
Although all the material in contact with blood has been used in other cardiac surgical procedures, patients continue to require anticoagulation. Patients are at risk of thrombotic or hemorrhagic stroke. Implanted blood pumps are powered by an external compressor weighing around six kg—much lighter than the former large console on wheels that in the past prevented hospital discharge. The weight of the device continues to be burdensome for patients, especially those who are more deconditioned. The sound produced by the device also is rather loud. Infections are a significant and relatively frequent complication of the TAH. The design element that contributes to this risk is the non-totally implantable design of the device with two wide drivelines tunnellized under the skin to deliver and withdraw driving compressed air to the implanted moving diaphragms. Unfortunately, the tube exit site is a possible entry point for bacteria, and therefore great care must be taken to minimize the risk of local and systemic infections.
A review of all the clinical data available in literature about CardioWest TAH is presented in Table 2.
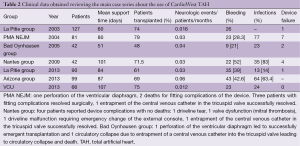
Full table
Despite the motor being implanted outside of the body, the complete heart prosthesis still weighs 160 g and displaces 400 mL of volume (9-11). This prevents the TAH from being implanted in many smaller patients, mostly women and adolescents. In 2013, the manufacturer received FDA designation as a HUD for the new 50 mL TAH to be used for DT and pediatric BTT. The 50 mL device is a smaller version of the 70 mL TAH and should fit in smaller patients that were previously underserved.
AbioCor TAH (Abiomed, Danvers, Massachusetts, USA) is the first artificial heart system that does not require percutaneous lines to be powered. Compared to the CardioWest TAH, the drive unit sits inside the patient’s body. The AbioCor TAH was first implanted in humans in 2001 (12,13). Fourteen patients have received the device in a DT trial for end-stage heart failure patients. Although all 14 patients in the initial group died, the longest survival time was over one year and a number of patients were able to be discharged home. After completion of the clinical trial, however, a post-market study was approved but only few cases were reported, CE mark was not pursued and the device has been practically unavailable since then (14).
AbioCor TAH consists of both external and internal components. The four internal components are the AbioCor thoracic unit, the battery, the controller, and the transcutaneous energy transfer (TET) coil. The thoracic unit houses two blood pumps separated by an energy converter. Each blood pump contains a flexible blood sac, which has inflow and outflow trileaflet valves, all made of a proprietary polyurethane (Angioflex). Hydraulic fluid fills the space between the blood sacs and the energy converter. When the hydraulic fluid is moved from one side to the other, the blood is squeezed out from one flexible chamber, thus mimicking systole; at the same time, blood is drawn into the other chamber, filling it for the next cycle, thus mimicking diastole. The left and right blood pumps alternately eject blood. A balance system promotes lower flow from the right blood pump than the left blood pump, to compensate for the left side of the heart receiving flow from the bronchial arteries. The power is transmitted to the energy converter from an external source by means of coupling external and internally implanted coils that receive radio waves and convert it into DC power. The implanted controller actuates the energy converter according to the set beat rate and balance system. By controlling the beat rate, cardiac output can be increased and right atrial pressure decreased; by controlling the balance system, left atrial pressure can be kept within an appropriate range. The implanted battery allows the patient freedom from external power sources for around half an hour.
Although this innovative design provides a solution to the risks of contamination from outside the body, the total weight of the implanted components is almost two kg and the minimum body surface area of recipients must be close to two m2: very few patients might accommodate such a large TAH. The presence of these bulky components might have a role in the observed high rate of infections (78% of the 14 clinical trial patients). Moreover, implantation is rather complex procedure: 2/14 patients did not survive surgery. The biocompatibility of materials and design of the implanted prosthesis did not achieve satisfactory results: 18 strokes were observed and cardiovascular accidents (CVA) were the primary cause of death in 43% of patients. No conclusion can be drawn on regarding the durability of implanted components and materials, since the average survival time was only 5.3 months after implantation. There were two device malfunction-related subject deaths. A subject died due to a membrane wearing out at 17 months, and a second subject died due to a motor-bearing stoppage at 4.8 months. The use of proprietary design and materials for prosthetic valves prohibits forecasting their reliability based on historical clinical use. Not only is AbioCor TET system a promising solution for decreasing the risk of infection, but it also improves patient quality of life, allowing completely un-tethered periods of operation. Nevertheless, it wastes approximately 30% of energy in transmission, thus requiring heavy portable batteries. The implanted TET coil is usually placed in a subclavicular position and the external coil must be stably secured on the patient’s skin: improper placement or positioning can cause thermal injury and/or patient discomfort.
The only Carmat TAH (Carmat, Velizy, France) was implanted in December 2013. It contains two ventricles, each with a blood compartment and a driving fluid compartment, separated by a flexible hybrid membrane. The hybrid membrane has a polyurethane layer at the fluid-contacting surface and bovine pericardial tissue on the blood-contacting surface (15,16).
Electro-hydraulic pumps create a systolic and a diastolic phase by moving the silicone fluid and deploying the hybrid membrane. The stroke volume and the beat rate of the prosthesis adapt automatically in response to changes in preload, detected by the pressure sensors located in the device. The resulting pulsatile blood flow ranges from 2 to 9 L/min with a flow adjustment on the right side to correct for the bronchial shunt. Bioprosthetic valves at the inlet and outlet of each blood compartment maintain the forward flow. The prosthesis is partially surrounded by a flexible compliance bag. A percutaneous driveline delivers power to the prosthesis and allows exchange of data and inputs.
The first and only patient to receive this device survived 75 days on support. The use of bioprosthetic valves and partial pericardium lining of the blood chambers are the most innovative features of this TAH. In vitro studies on samples of the hybrid membrane have shown limited consumption of fibrin, limited platelet adhesion, and minor blood cell depositions on the surfaces compared to positive controls. Even if the results need to be validated in the clinical study, a reduction of anti-coagulation therapy will likely be attainable. A patented method is used to secure the inlet valves of the TAH to the atrial cuffs once they have been sutured to the natural atria after heart excision, thus resulting in faster and safer implant procedure. Unfortunately, this implantable prosthesis is still quite large, it weighs 900 g; studies performed by the manufacturer claimed that it fit 65% of patients (86% of which were men).
In conclusion, biocompatibility of design and use of materials to decrease hemorrhagic and thromboembolic complications; reduced size of implantable and wearable components to fit in a broader patient population; and implementation of a fully implantable driving unit that is safe and fully operational without interruption of skin barriers continue to be limitations of the TAHs. The clinical utility of the TAH can be expanded once appropriate technological improvements are made.
TAHs: future perspectives
Much of the current research is focused on improving the TAH in order to overcome the problems mentioned above. Innovative biological tissue valves and coatings are under investigation. In our institution, we are investigating the use of decellularized pericardium to line TAH surfaces in contact with blood. Intermediate results on mechanical characterization and long term repopulation suggest the possibility of coating polyurethane membranes of different shapes (17-20).
Material science alone, however, cannot guarantee biocompatibility of a device without design optimization. Numerical simulations and flow visualization are mandatory for studying blood flow trajectories and design optimizations to facilitate more laminar blood flow. Turbulence can lead to platelet activation, thus increasing the risk of clotting (21).
There are a variety of projects focusing on the miniaturization of implanted drive unit of the TAH. Continuous-flow TAHs are favorable because of their simpler configuration, as there is no need for valves, or for the minimum stroke volume that makes any further downsizing impossible with traditional pulsatile TAHs. In addition, less mechanical components and moving parts result in less wear and failure points (22).
The Cleveland Clinic’s continuous-flow TAH has one motor and one rotating assembly supported by a hydrodynamic bearing. The right hydraulic output is self-regulated by passive axial movement of the rotating assembly to balance itself with the left output (23).
Bivacor is another TAH currently undergoing extensive research and development at the Texas Heart Institute. It consists of a single rotary pump that has only one moving part (the central impeller), which is totally suspended inside large clearances using magnetic levitation technology. It is small and it can simultaneously pump more than 8 L/min from both the left and right sides (24,25).
Despite the mentioned attractiveness of continuous flow TAH, nothing is known about tolerability of such a flow mode. In fact, there is a plethora of experience with continuous flow VADs showing that long term continuous flow MCS is very well tolerated; however, most VADs tend to maintain a small degree of pulsatility due to their filling from a pulsatile ventricle. Furthermore, some of them provide intermittent low speed periods when the native pulsatile ventricle pumps blood in parallel with continuous flow VAD.
In order to deliver pulsatile flow and to decrease drive unit size, ReinHeart TAH has been developed in Aachen, Germany (26,27). The actuator consists of a linear motor that displaces two pusher plates, alternatively compressing/releasing two flexible blood chambers (right and left artificial ventricles). Compared to the Abiocor and Carmat, there are no rotary pumps, no motion transduction and less mechanical components. The implanted drive unit and ventricles weighs around 500 g. A volume compensation chamber, a controller and a battery are implanted along with the unit. No implants in humans have been performed yet.
OneHeart TAH, a very attractive alternative represented by Padua Heart Project, is currently under development at our institution (Gerosa G, 2014, unpublished data). It is electromechanically driven; small in size due to its self-contained, implantable drive-unit, which consists of a linear motor with only one moving component; and it can deliver pulsatile or continuous blood flow. Its surface in contact with blood can be coated with decellularized pericardium in order to optimize biocompatibility. Further tests on hemodynamic performance and durability of design components are underway.
After the clinical experience with AbioCor, TET systems are rapidly being developed for VADs, and new implantable TAHs as well (28). HeartWare is in an agreement to develop and incorporate in their LVADs the Dualis MedTech (Seefeld, Germany) (29), a wireless technology based on the principle of inductive coupling (according to a press release). Leviticus Cardio (Givat Shmuel, Israel) uses a proprietary technology named coplanar energy transfer (CET) with two coils: a receiver coil, implanted next to the VAD and an external transmitter coil, worn around the chest so that the two coils are in the same plane (30). The transmitter coil encircles the receiver coil and inductively transfers electro-magnetic power to it from all directions, hence claiming a higher efficiency. Even more interesting is resonant electrical energy transfer, which allows power delivery to an implanted LVAD at larger distances (i.e., over room distances) without compromising mobility or requiring direct contact between the patient and the energy source. The FREE-D system, developed at Yale University, uses this high-efficiency resonant coupling technology. The transmitting and receiving resonators exchange energy by sharing magnetic fields that oscillate at a specific resonant frequency (31). Thoratec recently announced its collaboration with WiTricity (Watertown, MA, USA) for the development of a fully implantable ventricular assist system that also uses the resonant energy transfer principle (32).
TAHs require roughly more than twice as much energy to be delivered inside a patient’s body compared to the LVAD, thus requiring larger implanted coils and batteries. A compromise to limit the volume of implanted components while concurrently controlling the risk of infection might be a percutaneous power cable access steadily anchored to patient’s bone rather than to abdominal soft tissues, similar to the drive line post-auricular pedestal of Jarvik 2000 (Jarvik Heart, NY, USA) (33). In fact, this solution improves the patient quality of life by allowing them to bathe and shower, and seems to be more resistant to local infections (34).
Besides dedicated research, TAH might benefit from translational research from other seemingly unrelated fields. For example, small, high efficiency, long lasting batteries are developed in automotive research; and telemedicine can provide remote control and follow-up of patients. Only by pursuing TAH research targets in such a holistic and innovative manner can we accelerate the development of new artificial hearts and rapidly meet patients’ needs and expectations—“the future comes apace” (Shakespeare, Timon of Athens, Act II).
Acknowledgements
Disclosure: The authors declare no conflict of interest.
References
- Copeland JG, Smith RG, Arabia FA, et al. Cardiac replacement with a total artificial heart as a bridge to transplantation. N Engl J Med 2004;351:859-67. [PubMed]
- INTERMACS Interagency Registry for Mechanically Assisted Circulatory Support. Quarterly Statistical Report. 2013 4th Quarter. Available online: http://www.uab.edu/medicine/intermacs/statistical-summaries, accessed May 19, 2014.
- International Society for Heart and Lung Transplantation. Registries Slides. Available online: http://www.ishlt.org/registries, accessed May 19, 2014.
- Kalya A, Jaroszewski D, Pajaro O, et al. Role of total artificial heart in the management of heart transplant rejection and retransplantation: case report and review. Clin Transplant 2013;27:E348-50. [PubMed]
- Kirklin JK, Naftel DC, Kormos RL, et al. The Fourth INTERMACS Annual Report: 4,000 implants and counting. J Heart Lung Transplant 2012;31:117-26. [PubMed]
- Kirsch M, Mazzucotelli JP, Roussel JC, et al. Survival after biventricular mechanical circulatory support: does the type of device matter? J Heart Lung Transplant 2012;31:501-8. [PubMed]
- Torregrossa G, Morshuis MJ, Varghese R, et al. Results with Syncardia Total Artificial Heart Beyond 1 Year. ASAIO J 2014;60:626-34. [PubMed]
- Morris RJ. Total artificial heart--concepts and clinical use. Semin Thorac Cardiovasc Surg 2008;20:247-54. [PubMed]
- Slepian MJ, Alemu Y, Girdhar G, et al. The Syncardia (™) total artificial heart: in vivo, in vitro, and computational modeling studies. J Biomech 2013;46:266-75. [PubMed]
- Slepian MJ. The SynCardia Temporary Total Artificial Heart—Evolving Clinical Role and Future Status. US Cardiology 2011;8:39-46.
- SynCardia’s 50cc Total Artificial Heart Receives Two HUD Designations from the FDA for Destination Therapy and Pediatric Bridge to Transplant. Available online: http://www.syncardia.com/2013-press-releases/syncardias-50cc-total-artificial-heart-receives-two-hud-designations-from-the-fda-for-destination-therapy-and-pediatric-bridge-to-transplant.html, accessed May 19, 2014.
- Dowling RD, Gray LA Jr, Etoch SW, et al. The AbioCor implantable replacement heart. Ann Thorac Surg 2003;75:S93-9. [PubMed]
- Dowling RD, Gray LA Jr, Etoch SW, et al. Initial experience with the AbioCor implantable replacement heart system. J Thorac Cardiovasc Surg 2004;127:131-41. [PubMed]
- FDA Summary of Safety and Probable Benefit H040006 AbioCor Implantable Replacement Heart. Available online: http://www.accessdata.fda.gov/cdrh_docs/pdf4/H040006b.pdf, accessed May 19, 2014.
- Jansen P, van Oeveren W, Capel A, et al. In vitro haemocompatibility of a novel bioprosthetic total artificial heart. Eur J Cardiothorac Surg 2012;41:e166-72. [PubMed]
- Carmat website - Anatomical compatibility. Available online: http://www.carmatsa.com/index.php?option=com_flexicontent&view=items&cid=41&id=352&Itemid=42&lang=en, accessed May 19, 2014.
- Spina M, Ortolani F, El Messlemani A, et al. Isolation of intact aortic valve scaffolds for heart-valve bioprostheses: extracellular matrix structure, prevention from calcification, and cell repopulation features. J Biomed Mater Res A 2003;67:1338-50. [PubMed]
- Iop L, Renier V, Naso F, et al. The influence of heart valve leaflet matrix characteristics on the interaction between human mesenchymal stem cells and decellularized scaffolds. Biomaterials 2009;30:4104-16. [PubMed]
- Naso F, Gandaglia A, Bottio T, et al. First quantification of alpha-Gal epitope in current glutaraldehyde-fixed heart valve bioprostheses. Xenotransplantation 2013;20:252-61. [PubMed]
- Cigliano A, Gandaglia A, Lepedda AJ, et al. Fine structure of glycosaminoglycans from fresh and decellularized porcine cardiac valves and pericardium. Biochem Res Int 2012;2012:979351.
- Fritschi AJ, Laumen M, Spiliopoulos S, et al. Image based evaluation of mediastinal constraints for the development of a pulsatile total artificial heart. Biomed Eng Online 2013;12:81. [PubMed]
- Kobayashi M, Horvath DJ, Mielke N, et al. Progress on the design and development of the continuous-flow total artificial heart. Artif Organs 2012;36:705-13. [PubMed]
- Fumoto H, Horvath DJ, Rao S, et al. In vivo acute performance of the Cleveland Clinic self-regulating, continuous-flow total artificial heart. J Heart Lung Transplant 2010;29:21-6. [PubMed]
- Timms D, Fraser J, Thompson B, et al. Acute In-Vivo Trial of a Novel Rotary BiVAD/TAH. J Heart Lung Transplant 2009;28:S255-6.
- Greatrex NA, Timms DL, Kurita N, et al. Axial magnetic bearing development for the BiVACOR rotary BiVAD/TAH. IEEE Trans Biomed Eng 2010;57:714-21. [PubMed]
- Laumen M, Finocchiaro T, Cuenca E, et al. A Novel Total Artificial Heart for Destination Therapy: In-Vitro and In-Vivo Study. Biomed Tech (Berl) 2013. [Epub ahead of print].
- Pohlmann A, Lessmann M, Finocchiaro T. Drive optimisation of a pulsatile Total Artificial Heart. Arch Elect Eng 2011;60:169-178.
- Wang JX, Smith JR, Bonde P. Energy transmission and power sources for mechanical circulatory support devices to achieve total implantability. Ann Thorac Surg 2014;97:1467-74. [PubMed]
- HeartWare and Dualis MedTech Announce agreement to develop fully implantable ventricular assist system. Available online: http://ir.heartware.com/phoenix.zhtml?c=187755&p=irol-newsArticle&ID=1646139&highlight=, accessed May 19, 2014.
- Kassif Y, Zilbershlag M, Levi M, et al. A New Universal Wireless Transcutaneous Energy Transfer (TET) System for Implantable LVADs – Preliminary In Vitro and In Vivo Results. J Heart Lung Transplant 2013;32:S140-1.
- Waters BH, Smith JR, Bonde P. Innovative Free-Range Resonant Electrical Energy Delivery System (FREE-D System) for a Ventricular Assist Device Using Wireless Power. ASAIO J 2014;60:31-7. [PubMed]
- Thoratec announces development agreement with WiTricity for proprietary energy transfer technology. Available online: http://phx.corporate-ir.net/phoenix.zhtml?c=95989&p=irol-newsArticle&ID=1562263&highlight=Witricity, accessed May 19, 2014.
- Westaby S, Jarvik R, Freeland A, et al. Postauricular percutaneous power delivery for permanent mechanical circulatory support. J Thorac Cardiovasc Surg 2002;123:977-83. [PubMed]
- Gerosa G, Di Giammarco G, Sani G, et al. The Use of Post Auricular Pedestal Is a Winning Strategy in Reducing Driveline Infections during Long-Term Mechanical Support with LVADs. J Heart Lung Transplant 2011;30:S94.