Collateral network concept in 2023
Introduction
Spinal cord ischemia (SCI) in aortic surgery
SCI is rare (0.26% of all thoracic aneurysm repair) (1), but is the most serious complication after thoracic or thoracoabdominal aortic aneurysm (TAAA) repair, carrying a poor life expectancy regardless of type of procedure (one-year survival 63% in open repair, and 64% in endovascular repair) (2-5). In the original series of TAAA repair, Svensson et al. demonstrated the significant relation between the incidence and the extent of the aortic involvement using the Crawford classification of thoracoabdominal aneurysms and associated risk of paraplegia, where the more extensive type I (paraplegia or paraparesis, 15%) and II aneurysms (31%) that require removal of many of the sources of spinal cord blood supply are associated with the highest risk of perioperative neurological morbidity (2). Although the outcomes of TAAA repair have significantly improved over the past decades in experienced centers (paraplegia or paraparesis: Crawford I, 7.8%, and Crawford II, 13.9%) (4), the theory remains the same; the incidence of SCI increases with the removal of more aortic segments and branches of the descending to abdominal aorta, so-called segmental arteries (SAs). Below the cervical level, the SAs originate from the descending aorta, and consist of the intercostal arteries at the thoracic level and the lumbar arteries at the lumbar level. Understanding the anatomy and physiology of the blood supply to the spinal cord is paramount to performing TAAA repair (6-8). Intraoperative SCI can develop for several reasons; ischemia from intraoperative clamping, incomplete restoration of blood flow to the spinal cord, and embolism (2-4,9,10). On the other hand, there is another mechanism of temporary or permanent paraplegia/paraparesis, known as delayed SCI (4,10). Numerous strategies of spinal cord protection have been discussed which include: operative technique (staged operation, open vs. endovascular, extent of repair, revascularization of subclavian artery or internal iliac artery, distal aortic perfusion, hypothermia), management of SAs [coil embolization as a staged procedure, intentional endoleak during endovascular approach, direct SA perfusion including prevention of steal phenomena during open repair, reimplantation of intercostal arteries (ICAs)], hemodynamic status including collateral network concept [permissive hypertension, correction of anemia, cerebrospinal fluid (CSF) drainage, neuromonitoring], pharmacologic agents and so on (11-33). In this regard, cumulative laboratory studies and clinical observations suggest that a robust collateral network must exist to explain preservation of spinal cord perfusion when segmental vessels are interrupted (6-8,34,35). In this review, we will focus on the collateral network concept and its application.
Principles of collateral network concept
In 1881, Dr. Albert Adamkiewicz reported an artery originating from T8-L1, also known as the arteria radicularis magna or Adamkiewicz artery (AKA), as the principal vessel that feeds the lower thoracic, lumbar, and sacral portions of the spinal cord (36,37). Based on this theory, some groups have been trying to identify AKA by imaging modality and reimplant this to prevent SCI during TAAA repair. In a multi-center analysis from Japan utilizing preoperative computed tomography (CT) angiography, AKA was found in 97.6% of patients who underwent TAAA repair predominantly on the left side of SAs (36). However, reimplantation of these identified SAs did not eliminate paraplegia including delayed SCI. Importantly, the concept of AKA does not explain this pathophysiology (6-8,10,38). Furthermore, reconstruction of SAs does not seem essential to prevent paraplegia given the lower rate of SCI which has been demonstrated in endovascular repair (17-22,39). Other studies showed that SCI is correlated more with the number of intercostal pairs sacrificed rather than the sacrifice of a single, important blood supply such as the AKA (37,38).
In the 1990s, Dr. Griepp and colleagues proposed instead the existence of an extensive collateral network that supports spinal cord perfusion (Figure 1) (6-8,35,38,39). This collateral network includes an interconnecting complex of vessels in the intraspinal, paraspinous, and epidural spaces, and in the paravertebral muscles, including the intercostal and lumbar SAs as well as the subclavian and hypogastric arteries. In this concept, as opposed to the one major segmental input model (AKA), recognition of the importance of multiple inputs to the spinal circulation is paramount to preventing SCI. In a pig model, Etz et al. showed only a 50% paraplegia rate even after sacrificing all arteries which may support the additional blood supply to the spinal cord other than SAs (8). Another important result from Dr. Griepp’s group was that the incidence of SCI is correlated more with the number of intercostal pairs sacrificed rather than their location; more than 10 pairs or 8–12 pairs sacrificed beginning in the lower thorax (Th6 or below) showed a higher risk of SCI (37,40,41).
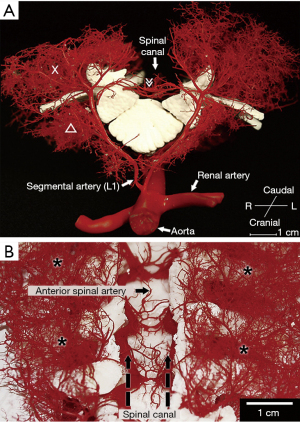
The maintenance of adequate spinal cord perfusion is crucial to the success of open and endovascular repair of extensive TAAA to prevent critical SCI when blood flow to the SAs is interrupted. Spinal cord perfusion pressure (SCPP) represents the net pressure gradient that drives oxygen delivery and blood supply to the cord, and is calculated as the difference between the collateral network pressure (CNP) and the pressure within the intrathecal space [CSF pressure » central venous pressure (CVP)] (6-8,13,42).
Clinically the mean arterial pressure (MAP) is a landmark of SCPP although MAP and SCPP are not equal. In an experimental model using a pig, Etz and colleagues demonstrated that CNP is 60–80% of MAP at baseline (39). When all SAs were taken in 2 stages a week apart, CNP fell only to 50% to 70% of baseline, and SCI was rare. In human beings, baseline CNP was 75% of mean aortic pressure, fell in proportion to the number of SAs ligated, and began recovery within 24 hours (39). Interestingly, they found that CNP was lower with non-pulsatile distal bypass than with pulsatile perfusion. Other experimental models by Geisbüsch et al. and Etz et al. showed that after experimental SA ligation, an early increase in collateral network flow of existing native vessels was observed for up to 48 hours, likely due to vasodilatation, followed by a definite increase in the number, density, and size of small arteries (Figure 2) (20,35). Furthermore, remodeling of paraspinous microvessels (arterioles up to 40 mm) was observed, not just in the density or the number of microvessels, but also in the spatial orientation of these vessels 5 days after completing SA occlusion (T4-L5) (20,35,40). These results suggest that the collateral network concept includes not only robust blood circulation to the spinal cord but also a dynamic remodeling process of the spinal cord perfusion where collateral supply is received from both from the cranial (the subclavian artery) and caudal (hypogastric arteries/internal iliac arteries) pathways to the area of the spinal cord that has been deprived of direct SA perfusion (Figure 3) (40).
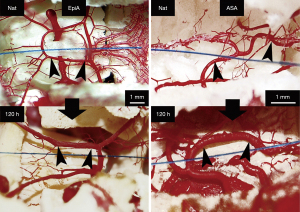
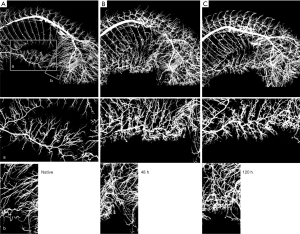
Clinical application of the collateral network concept
Staged approach and revascularization of the branches
In line with the dynamic remodeling process of increased spinal cord circulation after sacrifice of SAs, a staged repair strategy for extensive pathologies has emerged. Staged repair can be done by either open or endovascular approaches. Hybrid staged repair (open + endovascular) yields a lower incidence and less severity of SCI compared with single-stage repair (11,13). In patients with extensive TAAA involving proximal descending or arch pathology, open stage I arch repair, classic or frozen elephant trunk, can be used to facilitate stage II procedure by either open or endovascular repair including hybrid approach, to reconstruct visceral branches (14,43-48). Although hybrid or total endovascular arch repair is available, open arch repair remains a gold standard for aortic arch with extensive distal aortic pathology to provide a reliable proximal repair in this setting.
In Crawford type II TAAA repair, several staged approaches have been reported (12,13,27,28,43-48). Over the past decade, unique applications of the collateral network concept have emerged as staged approaches utilizing endovascular techniques (Figure 4). Minimally invasive segmental artery coil embolization (MISACE) prior to endovascular TAAA repair is a novel approach to reduce SCI (19-22); SA occlusion is performed using endovascular coiling to trigger arteriogenic preconditioning of the collateral network, thereby allowing for recruitment of otherwise redundant arterial collaterals to the spinal cord (19-22). A University of Leipzig group reported their initial experience and its efficacy (19). Utilization of MISACE is now under investigation as a randomized study, Paraplegia Prevention in Aortic Aneurysm Repair by Thoracoabdominal Staging (PAPA-ARTiS) trial (ClinicalTrials.gov Identifier: NCT03434314). Patient enrollment was closed in June 2022.
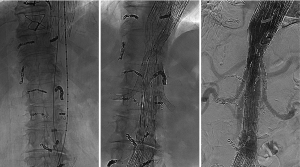
Temporary aneurysm sac perfusion as intentional non-completion of at least one stentgraft branch is expected to prevent complete aneurysm sac thrombosis and maintain spinal cord perfusion through patent intercostal and/or lumbar arteries (16,18,24,25,43). The delayed bridging stent method (temporary aneurysm sac perfusion) was reported by Kaspzac et al. in 2014 (Figure 5) (24). In this technique, a branched stentgraft is placed in a preliminary intervention, whereby a target vessel, usually the celiac artery, is not connected in order to maintain a temporary endoleak for the purposes of spinal cord perfusion. In the follow-up procedure, simultaneously to the sac perfusion branches, the procedure is completed by connecting the side branch to the target vessel. A similar concept was originally reported by Lioupis and colleagues in 2011 (25), known as the perfusion branch technique, where a branched custom-made endograft is made with a separate perfusion branch in the body of stentgraft to maintain a temporary “intentional” endoleak in order to perfuse the aneurysm and SAs followed by stage II endovascular completion. These novel methods are examples of clinical applications of the collateral network concept, and have demonstrated favorable SCI rates compared with conventional endovascular approaches covering the long aortic segment.
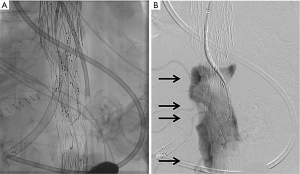
According to the collateral network concept, the left subclavian artery (LSCA) is an important collateral source via the internal mammary artery and other branches connected to the SAs (6-8). A meta-analysis by Huang and colleagues reported that revascularization of the LSCA is associated with a lower incidence of SCI after extensive coverage of SAs (44). This favorable outcome in the setting of Zone 1 or 2 thoracic endovascular aortic repair (TEVAR) with LSCA revascularization may suggest that revascularization of in situ SAs such as the anterior spinal artery is not necessary for SCI prevention. There are several options for revascularization of LSCA prior to TAAA repair: debranching the LSCA during open arch repair, left carotid artery to LSCA bypass, right axillary artery to left axillary artery bypass, or TEVAR (one-branch, fenestration, chimney, scalloped graft, etc.) (29,43-46). Recent data from the Society for Vascular Surgery Vascular Quality Initiative showed comparable outcomes between open and endovascular LSCA revascularization (29). Currently, LSCA revascularization is recommended for Zone 2 arch repair when patients have the following risk factors for SCI (30): (I) prior infrarenal aortic repair with occlusion of lumbar and middle sacral arteries; (II) planned long-segment (20 cm) coverage of the descending thoracic aorta where critical intercostal arteries originate; (III) hypogastric artery occlusion, and (IV) presence of early aneurysmal changes that may require subsequent therapy involving the distal thoracic aorta. A history of either repaired or unrepaired abdominal aortic aneurysm (AAA) is known as a risk of SCI during TAAA repair as lumbar or iliac arteries are occluded or narrowed in these patients (the number of patent SAs: 4.5±3.0 pairs in patients with AAA vs. 7.6±1.3 pairs in patients without AAA) (31).
The collateral network concept can also be applied to the extent of repair as surgical resection of life-threatening segments only may be an important strategy given the impact of paraplegia. According to the largest experience of open TAAA repairs by Dr. Coselli’s group, the incidence of SCI was lower in extent I or IV repair compared to extent II or III repair (4). For chronic aortic dissection with aneurysm cases, we previously reported that the growth rate of distal aorta is favorable after resection of most problematic segments (47). This concept is crucial especially in elective cases because most aneurysm surgeries are prophylactic (48).
Intraoperative blood flow to the SAs during TAAA repair
Steal phenomenon of the aorta or SAs has been recognized as a potential risk factor for SCI during TAAA repair (9,13). Shiiya and colleagues reported based on clinical experiences that aortic steal phenomenon was a major cause of intraoperative SCI, and some cases were reversible by clamping the SAs after recognizing neuromonitoring changes (9). Their findings support the importance of both the steal phenomenon and collateral network in demonstrating reversibility of intraoperative SCI by improving SCPP through clamping the SAs. Similarly, distal aortic perfusion while cross-clamping the aorta is another method to maintain SCPP (perfusion to the SAs) assisting spinal cord protection. A University of Texas group reported their experience of TAAA repair using the adjunct of distal aortic perfusion (increasing CNP) and CSF drainage (lowering CSF pressure), providing a low incidence of SCI (15). Recently, Haunschild and colleagues showed the significance of steal phenomenon even during distal aortic perfusion (49). In this report, distal aortic perfusion with blood flow steal from the SAs resulted in decrease in perfusion in the spinal cord regional flow, especially in the lower thoracic and upper lumbar levels (Th8-L2). Interestingly, this decrease of perfusion in the spinal cord increased when clamping the SAs. These results suggest that Th8-L2 are most sensitive to “segmental steal”, and segmental steal during TAAA repair using distal aortic perfusion also plays an important role in causing SCI.
Re-implantation of SAs during open TAAA repair has been discussed as a method of prevention of SCI. Dr. Coselli’s group reported their approach using left heart bypass with mild hypothermia (32–34 ℃, nasopharyngeal) where one or more pairs of intercostal and lumbar arteries were reattached, especially between T8 and L1, when possible (4). Some centers look for AKA as a preoperative evaluation of TAAA repair with a high rate of reimplantation rate (36). Dr. Estrera and Safi’s group reported the efficacy of neuromonitoring-guided reimplantation of SAs where patent important SAs (i.e., T8-T12) or adjacent patent SAs (T4-T7, L1, L2) were reimplanted if changes on somatosensory evoked potentials (SSEPs) and motor evoked potentials (MEPs) were encountered (43). A Japanese multi-center study looking at identification of AKA demonstrated that a risk of not reconstructing AKA segments was not significant in open repair, and whether AKA covered or not was not associated with SCI in endovascular approach (36). Furthermore, Dr. Griepp’s group reported a favorable SCI rate without any reimplantation of SAs based on the collateral network concept. Of note, they demonstrated that the number of SAs cut and location of SAs (number of sacrifice >8 with highest T6 or below) were associated with SCI (13,37,38,41).
Regardless of re-implanting SAs or not, the majority of aortic programs use some sort of approach based on the collateral network concept to prevent SCI such as increasing the MAP including distal aortic pressure, decreasing cerebrospinal fluid pressure, increasing hemoglobin levels by transfusion, or completion of repair to establish pulsatile flow to entire ‘‘collateral network” as soon as possible (4,15,36,37,42,43).
Importance of SCPP
Maintaining adequate SCPP is the key component of the collateral network concept to secure blood flow to the spinal cord. It is paramount to measure both upper body and lower body arterial pressure during TAAA repair. The MAP needs to be maintained on the high side (>90 mmHg) especially during sacrifice of the SAs and after completion of repair (2,6-8,39,47,49). This can be also achieved by increasing cardiopulmonary bypass flow through the femoral artery to increase a distal aortic perfusion pressure to the lower SAs (49). Care should be taken to maintain pulsatile flow of the upper body during partial cardiopulmonary bypass of the lower body as pulsatile flow provides more blood flow to the spinal cord, as demonstrated by Etz et al. (39).
Neuromonitoring such as MEP and/or SSEP is frequently used to detect early SCI during the procedure (4,23,37,42). Once SCI is suspected on MEPs or SSEPs after clamping SAs, the target MAP can be increased and measurement of MEP is repeated in 1 to 5 minutes to observe recovery of evoked potentials (13,14,43,47,49). Dr. Etz’s group from Leipzig introduced the efficacy of collateral network near-infrared spectroscopy (NIRS) (17,27) as non-invasive, real-time monitoring of spinal cord oxygenation which can be used during surgical repair and postoperatively. In a clinical series, lumbar collateral network NIRS oxygenation levels dropped significantly after proximal aortic cross-clamping but fully recovered after restoration of pulsatile flow to the baseline (17). They found that lumbar collateral network NIRS reacts to occlusion of SAs in real time and correlates with permanent neurologic deficit in animal models (27). Although further evidence is warranted, this approach has a potential as an adjunct to adjust intraoperative management such as increasing MAP or distal aortic perfusion pressure during anastomosis to increase SCPP.
Kawanishi et al. from Kobe University demonstrated that the duration of intraoperative hypotension after separation from cardiopulmonary bypass is an independent risk factor for SCI in patients with TAAA which supports the importance of maintaining SCPP (50). Etz et al. nicely demonstrated that SCPP is lower at completion of cardiopulmonary bypass or repair compared to baseline SCPP level (approximately one-third of the baseline) which returns to normal level by 120 hours postoperatively (39).
CSF drainage is another important adjunct to increase SCPP by reducing the CSF pressure/CVP. In a single-center randomized clinical study, Coselli and colleagues showed the efficacy of CSF drainage during open TAAA repair to reduce SCI (33). CSF drainage can also be used during endovascular repair for patients with risk factors for SCI such as long segmental coverage, occlusion of SAs or aortic branches, etc. (23,43-47). Dr. Estrera’s group reported the importance of protocol-based management of CSF drain and hemodynamics, known as CSF drain status/oxygen delivery/patient status (COPS) protocol (51). In this protocol, the goal parameters are: CSF drain pressure <5–10 mmHg, correcting oxygen saturation, goal hemoglobin >12 g/dL, cardiac index >2.5 L/min/m2, and MAP >90 mmHg with SCPP >80 mmHg.
Hypothermia
Mild hypothermia is frequently used as an adjunct of spinal cord protection during open repair, regardless of femoro-femoral bypass or left heart bypass (4,9,13,15). In an experimental model, Strauch and colleagues demonstrated the protective effect of mild hypothermia (32 ℃) over normothermia (36.5 ℃) which allows for 50 minutes of safe ischemic time of the spinal cord when cross-clamping the aorta (34). Deep hypothermia can be utilized in extensive TAAA repair expecting prolonged SCI during the procedure. Although there may be concern of complications related to deep hypothermia such as longer cardiopulmonary bypass time, bleeding, respiratory failure, renal failure, or infection, there are numerous benefits such as reliable organ protection including the spinal cord, no steal phenomenon, bloodless surgical field, and less complicated anastomosis without any clamping (4,36,43,52,53). Previous studies comparing open TAAA repair using deep hypothermic circulatory arrest versus beating hearts with warmer temperature (mild to moderate hypothermia) reported lower incidence of SCI utilizing deep hypothermic circulatory over mild to moderate hypothermia (52,53). In terms of ischemic tolerance of the spinal cord during deep hypothermia, Etz and colleagues studied the safe limit of lower body ischemic time using selective antegrade cerebral perfusion model during aortic arch repair, showing that 120 minutes of deep to low-moderate hypothermia (20–23 ℃) falls into the safe lower body ischemic time (54). Although their model was designed to assess the relationship between temperature and spinal cord protection during aortic arch surgery using antegrade cerebral perfusion, this result may provide significant insights into TAAA repair in terms of temperature management.
Delayed SCI
SCI can present in a delayed fashion with progressive lower extremity weakness (2,10,38). In the largest experience of 3,309 TAAA repairs from Dr. Coselli’s group, the proportion of delayed SCI (permanent paraplegia + paraparesis) was greater than immediate SCIs (delayed SCI, 55.6%, N=99/178 vs. immediate SCI, 44.4%, N=79/178) (4). They also reported a wide range of time frames regarding delayed SCI where paraplegia and paraparesis occurred 13 hours to 91 days postoperatively (10). In Dr. Griepp’s group’s experience, delayed SCI accounted for 85% of SCI after TAAA repairs where the breakdown of delayed SCI was further divided to intermediate-delayed (up to 48 hours postoperatively) and late-delayed SCI (>48 hours postoperatively) with almost equal distribution (38). A similar trend was observed in studies by Conrad et al. and Maeda et al. comparing open versus endovascular TAAA repair, where a greater percentage of patients showed delayed SCI in patients who received endovascular repair (55,56).
There are several potential mechanisms associated with delayed SCI, including unstable hemodynamics resulting in low SCPP, spinal cord edema, inflammatory response including ischemic-reperfusion injury, or occlusion of re-implanted SAs (2,9,10,38,57). Occurrence of delayed SCI itself strongly suggests the presence of the collateral network concept because acute occlusion or severe stenosis of one of the SAs or embolism is less likely as a cause of SCI in the postoperative period as a delayed onset. This late presentation of SCI also shows the importance of maintaining SCPP not only in the operative room but also in the intensive care unit to enhance collateral network circulation and its remodeling. Etz and colleagues analyzed delayed SCI patients and found that CVP was significantly higher in the paraplegic patients from 1 to 5 hours postoperatively compared to patients whose motor function recovered (38). Their result supports the importance of lower CSF pressure or CVP in the early postoperative period by utilizing CSF drainage to maintain sufficient SCPP. Although the duration of spinal drainage after TAAA repair is usually 24–48 hours after surgery (57), it needs to be determined based on individual SCI risk including the length of aorta replaced, the number of sacrificed SAs, patency of SAs, history of aortic surgery, or other factors as discussed above (4,28-30,37,38,57). When delayed SCI happens, several treatments are considered: vasopressors, systemic corticosteroids, naloxone, and osmotic agents (e.g., mannitol, glycerol), blood transfusion, and CSF drainage (10,38,57). In the largest experiences from the Texas Heart Institute, outcomes of delayed SCI were not significantly different compared to immediate SCI where both groups showed poor survival in the long-term (five-year survival was 31% in both groups) (10). According to the COPS protocol proposed by the University of Texas group, a CSF drain is maintained for 3 days given the onset of delayed SCI based on their experience (51). Cheung et al. reported increased chances of recovery of delayed SCI (5 out of 8 full recovery, and 3 had partial recovery) using the COPS protocol (57). As some delayed SCIs are reversible by appropriate treatment, frequent neurological evaluation and maintenance of SCPP is crucial after TAAA repair.
On the other hand, the role of routine insertion of CSF drain during TEVAR is unclear in terms of benefits and risks related to CSF drain insertion (intra cranial hypotension, intracranial hemorrhage, headache, local bleeding, and SCI related to spinal hematoma). A Duke University’s group reported the efficacy of a restrictive lumbar CSF drain algorithm in the setting of TEVAR for TAAA repair, where permissive hypertension and collateral revascularization showed 0% incidence of SCI (58). Of note, their algorithm is based on the collateral network concept.
Pharmacological effect on collateral network
Many pharmacologic options have been reported which include anti-inflammatories, antioxidants, antiapoptotic agents, drugs that reduce neuronal excitotoxicity, drugs that induce or mimic ischemic preconditioning, medications that reduce metabolic demand, and osmotic agents to reduce tissue swelling (59), but discussing all of these mechanisms would be beyond the purpose of our review. In terms of collateral circulation, systemic vasopressors (e.g., norepinephrine, epinephrine, vasopressin, or phenylephrine) are a common approach to increase the MAP although this causes vasoconstriction of the arteries, so it is important to maintain the balance between suitable MAP and appropriate use of vasopressors in the perioperative period.
Although clinical application of these drugs is not yet common, Svensson et al. reported the efficacy of topical approaches using intrathecal papaverine as a local vasodilator which results in lower SCI rate in patients with TAAA repair (SCI rate of intrathecal papaverine group, 3.6% vs. control, 7.5%) (60). The idea of this technique is to increase blood flow to the spinal cord according to the collateral network concept.
Conclusions
The collateral network concept includes not only the robust, anatomical blood supply to the spinal cord but also a dynamic, temporal and spatial remodeling process of the spinal circulation, and was suggested by Dr. Griepp and colleagues in 1990s based on both basic experiments and clinical experiences. This concept is now widely accepted when treating TAAA regardless of open endovascular or hybrid repair to prevent perioperative SCI.
Based on the current evidence available for the collateral network concept, the following recommendations for the management of spinal cord protection are proposed (Table 1): staged TAAA repair regardless of open or endovascular repair, if possible, resection of life-threatening segments only to minimize the length of repair, revascularization of the LSCA if it needs to be covered by stent graft during proximal repair, neuromonitoring during TAAA repair, CSF drainage if open repair or endovascular repair for long segment coverage, CSF pressure <12 mmHg for 48 hours after operation, maintaining high MAP (>90 mmHg) perioperatively, selective reconstruction of SAs with extensive aortic resection, avoidance of steal phenomenon of SAs, distal aortic perfusion during cross-clamping if indicated, mild or deep hypothermia depending on the extent of open repair, securing hemostasis to avoid unstable hemodynamics, and maintaining hemoglobin level >10–12 g/dL to maintain stable hemodynamics and oxygen delivery.
Table 1
Management | Considerations |
---|---|
Preoperative management | |
Surgical strategy | Staged procedure if possible for both open and endovascular repair |
Length of repair | Resection of life-threatening segments only |
Revascularization of the left subclavian artery | Yes |
Identification of the artery of Adamkiewicz by imaging study | Not necessary |
Intraoperative management | |
Neuro monitoring | MEP or SSEP |
Spinal drainage | Yes if patients have risks of SCI, open repair, or covering long segment TEVAR (>15–20 cm) |
MAP | >90 mmHg |
Cerebrospinal fluid pressure | <12 mmHg |
Segmental arteries | Ligation before opening the aneurysm with neuromonitoring |
Any change during clamping large SAs needs repeat MEP with increased MAP | |
Re-implantation of SAs is controversial but can be done with neuro monitoring guidance or intraoperative findings | |
Perfusion strategy (open repair) | Distal perfusion after distal anastomosis |
Selective perfusion of SAs is not necessary | |
Mild hypothermia (32 ℃) using partial bypass or deep hypothermic circulatory arrest | |
Postoperative management | |
Spinal drainage | Keep for 24–48 hours |
Drainage does not exceed 15–20 mL/hour to maintain CSF pressure or CVP <12 mmHg | |
Mean arterial pressure | >90–100 mmHg for first 7 days |
Neurological assessment | – |
Avoidance of anemia | Hemoglobin >10–12 g/dL |
MEP, motor evoked potential; SSEP, somatosensory evoked potential; SCI, spinal cord ischemia; TEVAR, thoracic endovascular aortic repair; SA, segmental artery; MAP, mean arterial pressure; CSF, cerebrospinal fluid; CVP, central venous pressure.
Acknowledgments
This article is dedicated to Dr. Randall B. Griepp, Emeritus Professor of Cardiothoracic Surgery at the Icahn School of Medicine at Mount Sinai, New York, USA. We all recognize and remember his contributions to the field of thoracic aortic surgery and collateral network concept.
Funding: None.
Footnote
Conflicts of Interest: The authors have no conflicts of interest to declare.
Open Access Statement: This is an Open Access article distributed in accordance with the Creative Commons Attribution-NonCommercial-NoDerivs 4.0 International License (CC BY-NC-ND 4.0), which permits the non-commercial replication and distribution of the article with the strict proviso that no changes or edits are made and the original work is properly cited (including links to both the formal publication through the relevant DOI and the license). See: https://creativecommons.org/licenses/by-nc-nd/4.0/.
References
- Gialdini G, Parikh NS, Chatterjee A, et al. Rates of Spinal Cord Infarction After Repair of Aortic Aneurysm or Dissection. Stroke 2017;48:2073-7. [Crossref] [PubMed]
- Svensson LG, Crawford ES, Hess KR, et al. Experience with 1509 patients undergoing thoracoabdominal aortic operations. J Vasc Surg 1993;17:357-68; discussion 368-70.
- DeSart K, Scali ST, Feezor RJ, et al. Fate of patients with spinal cord ischemia complicating thoracic endovascular aortic repair. J Vasc Surg 2013;58:635-642.e2. [Crossref] [PubMed]
- Coselli JS, LeMaire SA, Preventza O, et al. Outcomes of 3309 thoracoabdominal aortic aneurysm repairs. J Thorac Cardiovasc Surg 2016;151:1323-37. [Crossref] [PubMed]
- Blaisdell FW, Cooley DA. The mechanism of paraplegia after temporary thoracic aortic occlusion and its relationship to spinal fluid pressure. Surgery 1962;51:351-5.
- Griepp EB, Di Luozzo G, Schray D, et al. The anatomy of the spinal cord collateral circulation. Ann Cardiothorac Surg 2012;1:350-7. [Crossref] [PubMed]
- Etz CD, Kari FA, Mueller CS, et al. The collateral network concept: a reassessment of the anatomy of spinal cord perfusion. J Thorac Cardiovasc Surg 2011;141:1020-8. [Crossref] [PubMed]
- Etz CD, Homann TM, Luehr M, et al. Spinal cord blood flow and ischemic injury after experimental sacrifice of thoracic and abdominal segmental arteries. Eur J Cardiothorac Surg 2008;33:1030-8. [Crossref] [PubMed]
- Shiiya N, Wakasa S, Matsui K, et al. Anatomical pattern of feeding artery and mechanism of intraoperative spinal cord ischemia. Ann Thorac Surg 2009;88:768-71; discussion 772. [Crossref] [PubMed]
- Wong DR, Coselli JS, Amerman K, et al. Delayed spinal cord deficits after thoracoabdominal aortic aneurysm repair. Ann Thorac Surg 2007;83:1345-55; discussion 1355. [Crossref] [PubMed]
- O'Callaghan A, Mastracci TM, Eagleton MJ. Staged endovascular repair of thoracoabdominal aortic aneurysms limits incidence and severity of spinal cord ischemia. J Vasc Surg 2015;61:347-354.e1. [Crossref] [PubMed]
- Bischoff MS, Brenner RM, Scheumann J, et al. Staged approach for spinal cord protection in hybrid thoracoabdominal aortic aneurysm repair. Ann Cardiothorac Surg 2012;1:325-8. [Crossref] [PubMed]
- Etz CD, Zoli S, Mueller CS, et al. Staged repair significantly reduces paraplegia rate after extensive thoracoabdominal aortic aneurysm repair. J Thorac Cardiovasc Surg 2010;139:1464-72. [Crossref] [PubMed]
- Ohira S, Malekan R, Kai M, et al. Reoperative Total Arch Repair Using a Trifurcated Graft and Selective Antegrade Cerebral Perfusion. Ann Thorac Surg 2022;113:569-76. [Crossref] [PubMed]
- Estrera AL, Miller CC 3rd, Chen EP, et al. Descending thoracic aortic aneurysm repair: 12-year experience using distal aortic perfusion and cerebrospinal fluid drainage. Ann Thorac Surg 2005;80:1290-6; discussion 1296. [Crossref] [PubMed]
- Rosset E, Ben Ahmed S, Galvaing G, et al. Editor's choice--hybrid treatment of thoracic, thoracoabdominal, and abdominal aortic aneurysms: a multicenter retrospective study. Eur J Vasc Endovasc Surg 2014;47:470-8.
- Etz CD, von Aspern K, Gudehus S, et al. Near-infrared spectroscopy monitoring of the collateral network prior to, during, and after thoracoabdominal aortic repair: a pilot study. Eur J Vasc Endovasc Surg 2013;46:651-6. [Crossref] [PubMed]
- Youssef M, Salem O, Dünschede F, et al. Adjunct Perfusion Branch for Reduction of Spinal Cord Ischemia in the Endovascular Repair of Thoracoabdominal Aortic Aneurysms. Thorac Cardiovasc Surg 2018;66:233-9. [Crossref] [PubMed]
- Branzan D, Etz CD, Moche M, et al. Ischaemic preconditioning of the spinal cord to prevent spinal cord ischaemia during endovascular repair of thoracoabdominal aortic aneurysm: first clinical experience. EuroIntervention 2018;14:828-35. [Crossref] [PubMed]
- Geisbüsch S, Stefanovic A, Koruth JS, et al. Endovascular coil embolization of segmental arteries prevents paraplegia after subsequent thoracoabdominal aneurysm repair: an experimental model. J Thorac Cardiovasc Surg 2014;147:220-6. [Crossref] [PubMed]
- Etz CD, Debus ES, Mohr FW, et al. First-in-man endovascular preconditioning of the paraspinal collateral network by segmental artery coil embolization to prevent ischemic spinal cord injury. J Thorac Cardiovasc Surg 2015;149:1074-9. [Crossref] [PubMed]
- Addas JAK, Mafeld S, Mahmood DN, et al. Minimally Invasive Segmental Artery Coil Embolization (MISACE) Prior to Endovascular Thoracoabdominal Aortic Aneurysm Repair. Cardiovasc Intervent Radiol 2022;45:1462-9. [Crossref] [PubMed]
- Tenorio ER, Ribeiro MS, Banga PV, et al. Prospective Assessment of a Protocol Using Neuromonitoring, Early Limb Reperfusion, and Selective Temporary Aneurysm Sac Perfusion to Prevent Spinal Cord Injury During Fenestrated-branched Endovascular Aortic Repair. Ann Surg 2022;276:e1028-34.
- Kasprzak PM, Gallis K, Cucuruz B, et al. Editor's choice--Temporary aneurysm sac perfusion as an adjunct for prevention of spinal cord ischemia after branched endovascular repair of thoracoabdominal aneurysms. Eur J Vasc Endovasc Surg 2014;48:258-65. [Crossref] [PubMed]
- Lioupis C, Corriveau MM, Mackenzie KS, et al. Paraplegia prevention branches: a new adjunct for preventing or treating spinal cord injury after endovascular repair of thoracoabdominal aneurysms. J Vasc Surg 2011;54:252-7. [Crossref] [PubMed]
- Kotelis D, Geisbüsch P, Hinz U, et al. Short and midterm results after left subclavian artery coverage during endovascular repair of the thoracic aorta. J Vasc Surg 2009;50:1285-92. [Crossref] [PubMed]
- von Aspern K, Haunschild J, Ziemann M, et al. Evaluation of collateral network near-infrared spectroscopy during and after segmental artery occlusion in a chronic large animal model. J Thorac Cardiovasc Surg 2019;158:155-164.e5. [Crossref] [PubMed]
- D'Oria M, Kärkkäinen JM, Tenorio ER, et al. Perioperative Outcomes of Carotid-Subclavian Bypass or Transposition versus Endovascular Techniques for Left Subclavian Artery Revascularization during Nontraumatic Zone 2 Thoracic Endovascular Aortic Repair in the Vascular Quality Initiative. Ann Vasc Surg 2020;69:17-26. [Crossref] [PubMed]
- Matsumura JS, Lee WA, Mitchell RS, et al. The Society for Vascular Surgery Practice Guidelines: management of the left subclavian artery with thoracic endovascular aortic repair. J Vasc Surg 2009;50:1155-8. [Crossref] [PubMed]
- Baril DT, Carroccio A, Ellozy SH, et al. Endovascular thoracic aortic repair and previous or concomitant abdominal aortic repair: is the increased risk of spinal cord ischemia real? Ann Vasc Surg 2006;20:188-94. [Crossref] [PubMed]
- Martin DJ, Martin TD, Hess PJ, et al. Spinal cord ischemia after TEVAR in patients with abdominal aortic aneurysms. J Vasc Surg 2009;49:302-6; discussion 306-7. [Crossref] [PubMed]
- Miyamoto K, Ueno A, Wada T, et al. A new and simple method of preventing spinal cord damage following temporary occlusion of the thoracic aorta by draining the cerebrospinal fluid. J Cardiovasc Surg (Torino) 1960;1:188-97.
- Coselli JS, LeMaire SA, Köksoy C, et al. Cerebrospinal fluid drainage reduces paraplegia after thoracoabdominal aortic aneurysm repair: results of a randomized clinical trial. J Vasc Surg 2002;35:631-9. [Crossref] [PubMed]
- Strauch JT, Spielvogel D, Lauten A, et al. Importance of extrasegmental vessels for spinal cord blood supply in a chronic porcine model. Eur J Cardiothorac Surg 2003;24:817-24. [Crossref] [PubMed]
- Etz CD, Kari FA, Mueller CS, et al. The collateral network concept: remodeling of the arterial collateral network after experimental segmental artery sacrifice. J Thorac Cardiovasc Surg 2011;141:1029-36. [Crossref] [PubMed]
- Tanaka H, Ogino H, Minatoya K, et al. The impact of preoperative identification of the Adamkiewicz artery on descending and thoracoabdominal aortic repair. J Thorac Cardiovasc Surg 2016;151:122-8. [Crossref] [PubMed]
- Griepp RB, Ergin MA, Galla JD, et al. Looking for the artery of Adamkiewicz: a quest to minimize paraplegia after operations for aneurysms of the descending thoracic and thoracoabdominal aorta. J Thorac Cardiovasc Surg 1996;112:1202-13; discussion 1213-5. [Crossref] [PubMed]
- Etz CD, Luehr M, Kari FA, et al. Paraplegia after extensive thoracic and thoracoabdominal aortic aneurysm repair: does critical spinal cord ischemia occur postoperatively? J Thorac Cardiovasc Surg 2008;135:324-30. [Crossref] [PubMed]
- Etz CD, Zoli S, Bischoff MS, et al. Measuring the collateral network pressure to minimize paraplegia risk in thoracoabdominal aneurysm resection. J Thorac Cardiovasc Surg 2010;140:S125-30; discussion S142-S146. [Crossref] [PubMed]
- Geisbüsch S, Schray D, Bischoff MS, et al. Imaging of vascular remodeling after simulated thoracoabdominal aneurysm repair. J Thorac Cardiovasc Surg 2012;144:1471-8. [Crossref] [PubMed]
- Zoli S, Roder F, Etz CD, et al. Predicting the risk of paraplegia after thoracic and thoracoabdominal aneurysm repair. Ann Thorac Surg 2010;90:1237-44; discussion 1245. [Crossref] [PubMed]
- Etz CD, Di Luozzo G, Zoli S, et al. Direct spinal cord perfusion pressure monitoring in extensive distal aortic aneurysm repair. Ann Thorac Surg 2009;87:1764-73; discussion 1773-4. [Crossref] [PubMed]
- Estrera AL, Jan A, Sandhu H, et al. Outcomes of open repair for chronic descending thoracic aortic dissection. Ann Thorac Surg 2015;99:786-93; discussion 794. [Crossref] [PubMed]
- Huang Q, Chen XM, Yang H, et al. Effect of Left Subclavian Artery Revascularisation in Thoracic Endovascular Aortic Repair: A Systematic Review and Meta-analysis. Eur J Vasc Endovasc Surg 2018;56:644-51. [Crossref] [PubMed]
- Oderich GS, Ribeiro M, Hofer J, et al. Prospective, nonrandomized study to evaluate endovascular repair of pararenal and thoracoabdominal aortic aneurysms using fenestrated-branched endografts based on supraceliac sealing zones. J Vasc Surg 2017;65:1249-1259.e10. [Crossref] [PubMed]
- Heidemann F, Kölbel T, Kuchenbecker J, et al. Incidence, predictors, and outcomes of spinal cord ischemia in elective complex endovascular aortic repair: An analysis of health insurance claims. J Vasc Surg 2020;72:837-48. [Crossref] [PubMed]
- Ohira S, Malekan R, Kai M, et al. Aortic Reoperation After Prior Acute Type A Aortic Dissection Repair: Don't Despair the Repair. Ann Thorac Surg 2023;116:43-50. [Crossref] [PubMed]
- Geisbüsch S, Kuehnl A, Salvermoser M, et al. Increasing Incidence of Thoracic Aortic Aneurysm Repair in Germany in the Endovascular Era: Secondary Data Analysis of the Nationwide German DRG Microdata. Eur J Vasc Endovasc Surg 2019;57:499-509. [Crossref] [PubMed]
- Haunschild J, von Aspern K, Herajärvi J, et al. Impact of distal aortic perfusion on 'segmental steal' depleting spinal cord blood flow-a quantitative experimental approach. Eur J Cardiothorac Surg 2022;62:ezac213. [Crossref] [PubMed]
- Kawanishi Y, Okada K, Matsumori M, et al. Influence of perioperative hemodynamics on spinal cord ischemia in thoracoabdominal aortic repair. Ann Thorac Surg 2007;84:488-92. [Crossref] [PubMed]
- Estrera AL, Sheinbaum R, Miller CC, et al. Cerebrospinal fluid drainage during thoracic aortic repair: safety and current management. Ann Thorac Surg 2009;88:9-15; discussion 15. [Crossref] [PubMed]
- Carrel TP, Berdat PA, Robe J, et al. Outcome of thoracoabdominal aortic operations using deep hypothermia and distal exsanguination. Ann Thorac Surg 2000;69:692-5. [Crossref] [PubMed]
- Yoo JS, Kim JB, Jung SH, et al. Surgical repair of descending thoracic and thoracoabdominal aortic aneurysm involving the distal arch: open proximal anastomosis under deep hypothermia versus arch clamping technique. J Thorac Cardiovasc Surg 2014;148:2101-7. [Crossref] [PubMed]
- Etz CD, Luehr M, Kari FA, et al. Selective cerebral perfusion at 28 degrees C--is the spinal cord safe? Eur J Cardiothorac Surg 2009;36:946-55. [Crossref] [PubMed]
- Conrad MF, Ye JY, Chung TK, et al. Spinal cord complications after thoracic aortic surgery: long-term survival and functional status varies with deficit severity. J Vasc Surg 2008;48:47-53. [Crossref] [PubMed]
- Maeda T, Yoshitani K, Sato S, et al. Spinal cord ischemia after endovascular aortic repair versus open surgical repair for descending thoracic and thoracoabdominal aortic aneurism. J Anesth 2012;26:805-11. [Crossref] [PubMed]
- Cheung AT, Weiss SJ, McGarvey ML, et al. Interventions for reversing delayed-onset postoperative paraplegia after thoracic aortic reconstruction. Ann Thorac Surg 2002;74:413-9; discussion 420-1. [Crossref] [PubMed]
- Weissler EH, Voigt SL, Raman V, et al. Permissive Hypertension and Collateral Revascularization May Allow Avoidance of Cerebrospinal Fluid Drainage in Thoracic Endovascular Aortic Repair. Ann Thorac Surg 2020;110:1469-74. [Crossref] [PubMed]
- Ghincea CV, Ikeno Y, Aftab M, et al. Spinal Cord Protection for Thoracic Aortic Surgery: Bench to Bedside. Semin Thorac Cardiovasc Surg 2019;31:713-20. [Crossref] [PubMed]
- Svensson LG, Stewart RW, Cosgrove DM 3rd, et al. Intrathecal papaverine for the prevention of paraplegia after operation on the thoracic or thoracoabdominal aorta. J Thorac Cardiovasc Surg 1988;96:823-9.