Transcatheter aortic valve replacement for bicuspid aortic valve disease: does conventional surgery have a future?
Introduction
Bicuspid aortic valve (BAV) disease affects people worldwide as the most common congenital heart valve defect. Present in 2–4% of the population, BAV disease is associated with high rates and early onset of calcific aortic valve disease (CAVD), aortic insufficiency, and ascending aorta and/or aortic root dilation compared to tricuspid aortic valve patients (1-4). Historically, valve replacement has been with surgical aortic valve replacement (SAVR) however, in the past decade transcatheter aortic valve replacement (TAVR) as emerged as a safe and minimally invasive alternative. Currently, there is much debate on whether we can safely transition to TAVR for BAV patients due to the much more complex anatomy compared with tricuspid AV morphology patients. This review aims to provide an overview of the management of calcific aortic stenosis (AS) associated with BAV. This will include the risks and benefits or SAVR and TAVR and introduce strategies for reducing complications by utilizing 3D printing and computer modeling techniques.
BAV anatomy and classification
Due to the nature of congenital defects, the BAV anatomy has many different types that differ in the way the cusps are fused. The most commonly used BAV classification method was made by Sievers et al. and considers the specific cusps that are fused and the number of fused raphe (5). Recently, Jilaihawi et al. created a classification method more relevant to TAVR that considers the shape of the valve opening including tricommissural, bicommissural raphe, and bicommissural non-raphe types with coronary cusp fusion and mixed cusp fusion subtypes (6) (Figure 1). The length of fusion can also vary as the valve can have cusps that are partially fused. Common imaging modalities include high temporal resolution echocardiography, which can also provide hemodynamic information and high spatial resolution computed tomography (CT). Echocardiography alone has been shown to misdiagnose 88.5% of BAV patients as being tricuspid who were later diagnosed with BAV disease from cardiac CT (7). This stresses the need for routine high resolution CT imaging for planning valve replacements. However, even with CT imaging it can still be difficult to properly assess the fine structures of the anatomy due to the calcium blooming effect when a large amount of calcium is present.
BAV associated diseases and treatment
BAV disease is commonly associated with aortic valvular stenosis or insufficiency and aortic aneurysms. The following sections will outline these common diseases associated with BAV disease and the available treatment options.
Aortic stenosis
AS is mainly caused by CAVD, which is a degenerative disease involving the growth of calcific lesions within the soft tissue. CAVD is commonly associated with BAV disease—roughly 50% of the population of people effected by CAVD also have BAV disease (2). CAVD is also known to have an earlier onset in BAV patients compared to tricuspid patients—many of them are younger and have lower surgical risk (8). Often BAVs have elliptically shaped openings and heavily calcified raphes making the anatomy considerably more complicated compared to tricuspid patients. Treatment for CAVD is through full replacement of the valve and historically has been through SAVR consisting of surgical excision of the native tissue and implantation of a bioprosthetic or mechanical valve. However, the field is continually transitioning to TAVR due to positive clinical comparisons to SAVR and fast patient recovery times. Recent clinical trials have shown similar outcomes between SAVR and TAVR after five years in tricuspid aortic valve patients leading to Food and Drug Administration (FDA) approval of TAVR for low surgical risk patients (9,10). Some clinical data exists showing similar outcomes between tricuspid and BAV patients which led to FDA removal of the cautionary label of TAVR use in BAV patients (11,12). However, these studies were not long term and tended to be very patient selective by not encompassing the complex anatomy BAV cases. As of 2020, ~9% of TAVR cases are for BAV patients (7). This number is expected to rise yet there has not been a comprehensive clinical trial comparing TAVR for tricuspid and TAVR for BAV low surgical risk patients. Yoon et al. showed that the presence of the calcified raphe and excess leaflet calcification increases the risk of aortic root rupture and paravalvular regurgitation (PVR) (13) (Figure 2). The difference in outcomes with respect to variance in BAV anatomy makes the TAVR for BAV patient selection process very important.
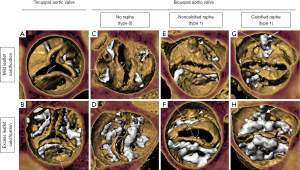
Aortic insufficiency and aortopathy
Aortic insufficiency is defined by improper closing of the aortic valve cusps causing regurgitation during diastole. Aortic insufficiency has been found to be present in 47% of BAV patients at the time of BAV diagnosis (3). Additionally, aortopathy is known to be present in roughly 50% of BAV patients (4). Vessel dilation is classified by the location of dilation; type 1 is dilation of the ascending aorta, type 2 is dilation of the aortic arch and type 3 is dilation of the aortic root (4). Aortic insufficiency in BAV patients can be caused by aortic dilation, cusp prolapse, or cusp restriction from the raphe (14). Treatment strategy depends on the cause of insufficiency—aortic dilation requires replacement of the ascending aorta and/or the aortic root and cusp prolapse/restriction can commonly be repaired by resecting the raphe (14). Additionally, when the cause of insufficiency is aortic root dilation it is not necessary to replace the valve as valve sparing root replacement has been shown to be safe and effective regardless of the severity (15). Although TAVR has been performed in some high- and extreme-risk patients with severe aortic insufficiency, the results have not been favorable and is not recommended.
TAVR adverse outcomes: concern in complex BAV anatomies
TAVR was originally applied in patients with tricuspid aortic valve morphologies with commonly symmetric root anatomy and calcific burden. However, BAVs are commonly elliptical and their diameter is not always constant with respect to height (16). Additionally, the calcium distribution can be more complex with the presence of a calcified raphe. The following sections will outline the common complications following TAVR and their relevance to BAV patients including PVR, aortic root rupture, valve leaflet thrombosis, increased permanent pacemaker implantation (PPI), coronary access and durability.
PVR and aortic root rupture
PVR is one of the most common complications associated with TAVR and is defined as backwards blood flow from the aorta to the left ventricle during diastole due to improper device sealing and, when moderate to severe, has been associated with increased patient mortality (17). Stent undersizing, high calcium volume, stent eccentricity and unfavorable positioning are all causes of PVR (18-20). Specifically, this is one of the most common complications following TAVR in those with a bicuspid AV as the annulus in these patients is eccentric and can have high volumes of calcium. Proper CT analysis and oversizing of the TAVR prosthesis may mitigate PVR (21,22). The most dreaded complication remains an aortic root rupture following a balloon-expandable device or post-dilation in a self-expandable device deployment and has an increased risk of occurring when high volumes of calcium are present. Aortic root rupture is a result of excess damage to the native tissue from calcium protrusion (23). BAV patients with high calcium volume and a calcified raphe have the highest risk of rupture (4.5%) as opposed to BAV patients with low calcium volume (0.9%) (13). A common preventative strategy is for the operator to reduce the balloon filling volume as to reduce the stress on the native tissue (23). However, this increases the risk of PVR. The decision between residual PVR or root rupture remains the most important and controversial aspect of TAVR in BAV patients.
Currently, it is very difficult to determine the risk of aortic root rupture other than analyzing the calcium severity. This is due to high patient variability in calcium distribution where calcium can be present on the cusp free edges, at the aortic annulus and in the left ventricular outflow tract making it difficult to determine which calcific nodules may puncture the tissue. Additionally, the patient tissue compliance has a significant amount of variability, most closely associated with age, making it very difficult to know how much stress is needed to rupture the tissue (24). Balloon volume is mostly determined procedurally where the operator may stop increasing volume if they feel too much resistance from the native tissue which may leave the patient with PVR. These counteracting strategies make it difficult to avoid moderate to severe PVR and aortic root rupture in TAVR for complex BAV patients, and determine the risk of such complications prospectively due to the complex nature of the anatomy (Figure 3). Ultimately, BAV patients with high amounts of calcium should be strongly considered for SAVR if they are low surgical risk, and if TAVR is necessary, a degree of caution should be used.
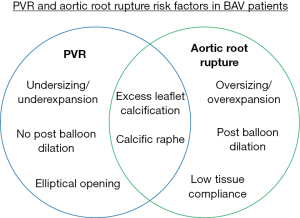
Thrombosis and durability
Thrombosis and valve durability are very important in the low surgical risk population as they serve to benefit more from a longer valve lifespan as to avoid re-intervention. Leon et al. found higher thrombosis rates following TAVR (2.6%) compared to SAVR (0.7%) and similar valve deterioration after two years in low surgical risk tricuspid patients (25). Valve thrombosis normally forms in the neo-sinus, which is the pocket located between the bioprosthetic cusp and the native cusp, and is likely caused by reduced blood flow in this region (26-29). Valve durability is thought to be related to the stress on the native leaflets which is dependent on the geometry of the deployed stent and the hemodynamics of the device (30-32). Due to the complex dynamic nature of the TAVR deployment it is common for the stent to be asymmetrical post procedure. This includes stent ellipticity, under expansion, and tilt with respect to the native sinus (29,33,34). Each of these asymmetrical deployment types have been associated with adverse hemodynamic environments demonstrated in three separate studies (Figure 4) (35-37). Stent eccentricity was found to create localized regions of high Reynolds shear stress and increased turbulence intensity (35). Under-expanded deployments were found to increase bioprosthetic leaflet folding and increase neo-sinus blood residence time (36). Tilted stent deployment with respect to the native sinus was found to increase the fluid residence in the sinus that the stent was angled away from (37). Each of these hemodynamic environments could increase thrombosis risk and lower device durability.
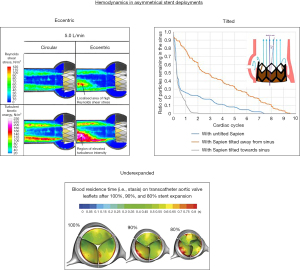
Asymmetric deployment is caused by reduced balloon volume, native valve elliptical opening and increased calcium volume which is of particular concern for anatomically complex BAV patients who have elliptical openings, high calcium volumes, and often have reduced balloon volume deployments. Overall, BAV patients are more predisposed to asymmetrical deployments and adverse hemodynamic environments with use of TAVR. This indicates thrombosis and valve deterioration will likely be a concern long-term. However, because long-term data on TAVR for BAV is very limited it is not certain thrombosis and durability will be a concern and requires additional long-term follow-up clinical studies.
Pacemaker implantation
PPI is one of the most common complications following TAVR. PPI is caused by interaction between the stent frame and/or the catheter with the conduction system located below the membranous septum which is located between the right and non-coronary sinuses (38,39). It is associated with device type, deployment depth, oversizing/overexpansion, membranous septum length, preexisting conduction abnormalities and severity of AS (38,40). It is also less common in younger healthy individuals (38). However, PPI occurrence can have increased consequences in young patients as it has various long-term adverse effects on the heart (38). Thus, PPI risk should be considered when evaluating young low surgical risk BAV patients for TAVR vs. SAVR as deploying above the membranous septum, using a balloon-expandable TAVR and avoiding oversizing can help mitigate PPI risk.
Coronary obstruction and access
Coronary access is always a concern during TAVR patient selection. This is especially true in young patients when considering their lifetime management of AS. Coronary obstruction is associated with low coronary heights and small sinus of Valsalva diameters (41). BAV patients have been found to have larger sinus of Valsalva diameters and no difference in coronary heights compared to tricuspid patients (8). In some BAV patients the coronary ostium location can be abnormal and located close to the native commissure where in combination with heavy calcification can decrease the gap between the native tissue and the coronary ostium following TAVR. When considering secondary replacement, it is important to consider the type and position of the implant. TAVR deployment in SAVR has a preventative strategy for coronary obstruction known as BASILICA which involves intentional laceration of the bioprosthetic leaflet allowing for blood flow to the coronary artery (42). However, if TAVR is performed as the first intervention it can increase the risk of coronary access issues following the second TAVR procedure, especially if the first valve was a self-expanding prosthesis that had supra-annular leaflets. The supra-annular bioprosthetic cusp suturing for the self-expandable device places the bioprosthetic cusps higher up on the frame and closer to the coronary ostium. Similarly, increased deployment heights also place the bioprosthetic cusps closer to the coronary ostium. BASILICA has been shown to be much less effective for TAVR in TAVR (43). When evaluating young low surgical risk BAV patients for TAVR vs. SAVR the height and location of the coronary artery should be measured and the risk of coronary obstruction following the first and second intervention should be considered.
SAVR risks and benefits
SAVR for the management of AS has its inherent risks and benefits. The main risks are associated with the physically demanding nature of the procedure where rates of death, stroke, or rehospitalization of 17.4% have been seen in low surgical risk tricuspid patients after two years (25). The short-term recovery time and possibility of psychological damage following open heart surgery is also a factor when a patient is considering intervention. However, in anatomically complex BAV patients the main benefit of SAVR is significantly reducing the common risks associated with TAVR that arise from the elliptical, heavily calcified anatomy including aortic root rupture, PVR, and asymmetrical deployments. The elliptical native leaflets and heavy calcification are excised making it a non-factor in the replacement. In young patients where future coronary access is a concern for repeated intervention, SAVR also has the benefit of the BASILICA technique which allows for blood flow to the coronary artery and is not as effective for TAVR in TAVR procedures (42,43). A secondary benefit of SAVR is it allows the parallel replacement of a dilated aortic root and/or aorta which is very relevant in BAV patients due to the common association of valvular and aortic disease. Lastly, in the case of aortic insufficiency, surgery can repair the malfunctioning native valve and restore it to proper function without the need for replacement. Overall, in complex BAV anatomies the risks of TAVR outweigh the risks and physically demanding nature of SAVR in low surgical risk patients where the surgical device will likely have less adverse complications and have increased durability, however, this has yet to be validated with a clinical trial comparing TAVR vs. SAVR in low surgical risk BAV patients.
Optimal TAVR for BAV strategy
In patients who are high or extreme surgical risk where TAVR is often the only option, the effectiveness of various TAVR strategies and when they should be used can be a difficult decision. The operator has the choice between balloon-expandable or self-expandable devices and can alter the balloon filling volume and device placement and can post dilate the stent in either device. Each of these choices can impact the risk for PVR, aortic root rupture, pacemaker implantation and the device hemodynamics.
Use of balloon-expandable device and post balloon dilation
In severely calcified BAVs, self-expandable devices often result in the stent being very deformed causing improper opening and closing of the bioprosthetic leaflets and PVR. The use of a balloon-expandable device or post dilating with a balloon can help to mitigate PVR (19,20). Of course, these strategies should be used with caution in high calcium volume patients where there is risk of aortic root rupture. Currently there is no preventative mechanism to stop balloon expansion prior to damaging the tissue and is completely up to the operator’s experience to stop if they feel strong resistance from the tissue. Ultimately, PVR can be decreased with balloon expansion, but should be performed with caution as to not damage the native tissue.
Importance of deployment depth
The deployment depth for balloon-expandable valves is often intra-annular—that is to deploy the stent just below the native annulus as to have the bioprosthetic leaflets be at the same depth as the native leaflets. However, this may not always be optimal especially for heavily calcified BAV patients who may have a circular annulus and an elliptical leaflet opening which can lead to anchoring issues (16). For self-expandable valves, the deployment depth can affect the risk of pacemaker implantation where a decrease in deployment height can increase the risk of contacting the inferior border of the membranous septum which is the location of part of the conduction system (44). Measuring the membranous septum length preoperatively can assist in determining deployment depth with respect to pacemaker implantation risk. Coronary access should also be considered when deciding the deployment depth in young patients where repeated intervention may be necessary. Ensuring the top of the bioprosthetic cusps are below the coronary ostium can help prevent future coronary obstruction or coronary access issues. Overall, the deployment depth is an important consideration for device anchoring, PPI, and coronary access.
Improving TAVR for BAV procedural strategies and planning
Given many BAV patients are low surgical risk, they often have the choice of TAVR or SAVR. TAVR outcomes are very dependent on the patient anatomy making the patient selection an extremely important decision especially when considering patient lifetime management in young patients. It is known that increased native valve resistance from the raphe and calcium volume increases PVR, aortic root rupture, and asymmetrical stent occurrence however, refined cutoff points and strict guidelines have not been established. This is mainly attributed to the lack of structured clinical trials assessing TAVR vs. SAVR for BAV safety. These factors make it difficult to evaluate the risk of TAVR during the patient planning process. The following sections highlight new procedural TAVR strategies for mitigating TAVR associated risks and the state of 3D printing and computer modeling technologies to aid in procedural planning.
New procedural strategies for complication prevention
Aortic root rupture is a very random event and is dependent on many different factors that are not measurable with medical imaging, making it very difficult to predict. Preventative techniques are to reduce balloon filling volume based on the operator’s sense of the tissue resistance and planned volume reduction based on observed high calcium volume. Snir et al. recently evaluated the feasibility of calculating tissue stress from balloon pressure and using it as a guide to prevent root rupture (45). This is a step in the right direction however, it does not capture high local stresses from calcium piercing into the tissue as the pressure measurement is measured globally throughout the balloon and does not factor patient specific material properties and thickness.
Manufacturers have recently added larger skirts and stent covers to their devices in the SAPIEN 3 Ultra and Evolut PRO+ which contribute to PVR reduction. Concerning PPI, some studies have shown rates as low as 3% through careful consideration of device placement with respect to the conduction system and alternate fluoroscopic views to allow for optimal positioning (46,47). Device under-sizing is common in BAV patients who have cone shaped valve openings. Preventative strategies have been developed consisting of balloon pre-dilation and visualizing the diameter of the balloon along the height of the valve and measuring the valve diameter at various locations other than the annulus (16,33). As for preventing asymmetrical deployments in BAV patients, preventative strategies have not yet been developed other than the use of balloon dilation.
Procedural planning: 3D printing
Benchtop analysis in the past has mostly contributed by providing more detailed information on valve and device structural and hemodynamic function on a more fundamental level. However, recently 3D printing has made strides in its resolution and non-uniform material property printing capabilities. This has allowed for accurate reconstruction of patient’s anatomy from CT and can give valuable insight for procedural planning (48). The main benefits are that it provides a 3D visual of the anatomy for the operator and has even shown correlation between measured geometric gaps to observed PVR in balloon-expandable and self-expandable valves (49,50).
One of the biggest challenges in 3D printing is the material properties. 3D printed soft materials are often very fragile and the material is not patient specific, making it difficult to accurately model structural deformation for assessing device deployment geometry and performing proper hemodynamic assessment in a flow loop. Additionally, there are very high amounts of resources required. The process is very time-consuming consisting of manual computer reconstruction of the anatomy, long printing times of up to multiple days, many hours of tedious cleaning of the support material, expensive 3D printing materials, machines, engineering software and trained engineer labor hours. Overall, 3D printing can accurately capture the geometry of the anatomy and can provide some geometric predictions of the procedure but currently has material property and resource efficiency limitations preventing it from widespread use.
Procedural planning: computer modeling
Computer simulation for prospective planning has been developing over the past decade. Many groups have developed accurate structural models for simulating the deployment of both balloon and self-expandable valves (51,52). This has been shown to have better predictive power for coronary obstruction compared to traditional methods (53). PPI has been shown to be accurately predictable for self-expandable valves in BAV anatomies based on the contact area between the stent and the inferior border of the membranous septum (54). Computational fluid dynamics has also been shown to accurately predict PVR severity in self-expandable and balloon-expandable TAVs (52,54-56). The prediction model process for balloon-expandable valves is shown in Figure 5 including anatomical reconstruction, stent deployment, and risk assessment for coronary obstruction and PVR.
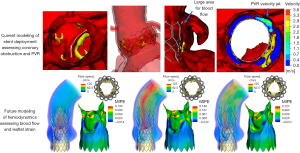
Originally these models were very resource expensive including many manual hours for skilled engineers, expensive software, and long simulation times. However, the models are becoming more efficient through the incorporation of artificial intelligence and machine learning. This includes nearly automatic computer reconstruction of the patient anatomy, reduced order simulation models for the deployment of the stent, and machine learning risk assessment (57-59). This has enabled commercial availability of prospective modeling including companies such as HeartFlow offering coronary artery stenting modeling, FEops offering TAVR and left atrial appendage occluder modeling, and DASI Simulations with transcatheter aortic and mitral modeling. These companies to date have modeled thousands of patients to assist in patient planning.
Medical imaging currently cannot provide information on the vessel thickness, intricate geometric calcium integration, tissue fiber distribution, and residual tissue stress from physiological loading. Geometric factors are particularly complex in BAV patients often due to increased calcium volume, abnormal fiber distribution due to the nature of the congenital defect (60), and difficulty to assess the precise length and degree of fusion at a raphe. These factors make it very difficult to accurately measure the stress in the native tissues to assess for possible tissue damage. Currently, most TAVR models do not incorporate both structural deformation and fluid responses together, known as fluid-structure interaction, which is very difficult to simulate. It would be very advantageous clinically to simulate the opening and closing of the device for assessing pressure gradient and fluid stagnation regions, which provide information on the device function and durability, especially in young patients being evaluated for TAVR.
Fluid-structure interaction models are in their earlier stages where some existing models simulate the opening and closing of the device leaflets in the patient specific anatomy, however these all have their limitations (57,61-63) (Figure 5). Mainly, the geometric simplification of the anatomy, rigid vessels, and very long simulation times up to multiple weeks. Overall, computational modeling is already being used to aid in clinical planning and has shown accuracy in predicting PVR, PPI, and coronary obstruction. However, modeling has increased limitations for simulating complex anatomies, especially BAVs, due to the abnormal geometry and unknown patient specific properties making it difficult to assess aortic root rupture. Additionally, thrombosis and long-term durability assessment is difficult due to the high complexity and low efficiency of fluid-structure interaction simulations.
Conclusions
TAVR has changed the field of valve replacement and is currently projected to increase in use for BAV patients with AS over SAVR due to fast patient recovery times and recent FDA approval for low surgical risk patients and removal of the TAVR for BAV precautionary label. It is known that calcification levels and complex BAV anatomical structures contribute to increased complication risks following TAVR making SAVR the more optimal choice in anatomically complex low surgical risk BAV patients. SAVR also has advantages when considering dual treatment of aortopathy which is common in BAV patients. Cutoffs for acceptable TAVR in BAV patients based on calcium volumes and distributions is an area of active investigation. Additionally, the long-term side effects from asymmetrical stent deployments associated with TAVR for BAV patients are unknown. Future studies including a long-term clinical trial evaluating the differences between TAVR and SAVR in low surgical risk BAV patients can assist in filling the knowledge gaps for a safer transition to TAVR. New patient screening techniques such as 3D printing and computer modeling can provide more information on the suitability for highly calcific BAV patients for TAVR by predicting the risk of PVR, PPI, and coronary obstruction however, the determination of their effectiveness in their predictability of aortic root rupture, thrombosis, and durability requires further studies.
Acknowledgments
Funding: None.
Footnote
Conflicts of Interest: BBY has a patent pending as co-inventor of patents related to computational predictive modeling of heart valves. PKY: consultant for Edwards Lifesciences, Medtronic Inc., Abbott Vascular, Shockwave Medical. VHT: consultant or research with Abbott Vascular, Boston Scientific, Cryolife, Edwards Lifesciences, Medtronic Corp., and Shockwave Medical and stakeholder in Dasi Simulations. LPD: stakeholder in Dasi Simulations, and has a patent pending as co-inventor of patents related to computational predictive modeling of heart valves.
Open Access Statement: This is an Open Access article distributed in accordance with the Creative Commons Attribution-NonCommercial-NoDerivs 4.0 International License (CC BY-NC-ND 4.0), which permits the non-commercial replication and distribution of the article with the strict proviso that no changes or edits are made and the original work is properly cited (including links to both the formal publication through the relevant DOI and the license). See: https://creativecommons.org/licenses/by-nc-nd/4.0/.
References
- Siu SC, Silversides CK. Bicuspid aortic valve disease. J Am Coll Cardiol 2010;55:2789-800. [Crossref] [PubMed]
- Yutzey KE, Demer LL, Body SC, et al. Calcific aortic valve disease: a consensus summary from the Alliance of Investigators on Calcific Aortic Valve Disease. Arterioscler Thromb Vasc Biol 2014;34:2387-93. [Crossref] [PubMed]
- Michelena HI, Desjardins VA, Avierinos JF, et al. Natural history of asymptomatic patients with normally functioning or minimally dysfunctional bicuspid aortic valve in the community. Circulation 2008;117:2776-84. [Crossref] [PubMed]
- Verma S, Siu SC. Aortic dilatation in patients with bicuspid aortic valve. N Engl J Med 2014;370:1920-9. [Crossref] [PubMed]
- Sievers HH, Schmidtke C. A classification system for the bicuspid aortic valve from 304 surgical specimens. J Thorac Cardiovasc Surg 2007;133:1226-33. [Crossref] [PubMed]
- Jilaihawi H, Chen M, Webb J, et al. A Bicuspid Aortic Valve Imaging Classification for the TAVR Era. JACC Cardiovasc Imaging 2016;9:1145-58. [Crossref] [PubMed]
- Kim WK, Liebetrau C, Fischer-Rasokat U, et al. Challenges of recognizing bicuspid aortic valve in elderly patients undergoing TAVR. Int J Cardiovasc Imaging 2020;36:251-6. [Crossref] [PubMed]
- Philip F, Faza NN, Schoenhagen P, et al. Aortic annulus and root characteristics in severe aortic stenosis due to bicuspid aortic valve and tricuspid aortic valves: implications for transcatheter aortic valve therapies. Catheter Cardiovasc Interv 2015;86:E88-98. [Crossref] [PubMed]
- Mack MJ, Leon MB, Thourani VH, et al. Transcatheter Aortic-Valve Replacement with a Balloon-Expandable Valve in Low-Risk Patients. N Engl J Med 2019;380:1695-705. [Crossref] [PubMed]
- Popma JJ, Deeb GM, Yakubov SJ, et al. Transcatheter Aortic-Valve Replacement with a Self-Expanding Valve in Low-Risk Patients. N Engl J Med 2019;380:1706-15. [Crossref] [PubMed]
- Edwards Lifesciences Lsasuthv. Approval for modifying the labeling to remove the precaution regarding patients with a congenital bicuspid aortic valve. In: Administration FaD, editor. 2020. Available online: https://www.accessdata.fda.gov/scripts/cdrh/cfdocs/cfpma/pma.cfm?id=P140031S107
- Medtronic Corevalve LLC. Medtronic CoreValve Evolut R System MCEPS, and Medtronic Evolut PRO+ System. Approval for modifying a precaution in the labeling regarding patients with a congenital bicuspid aortic valve. In: Administration FaD, editor. 2020. Available online: https://www.accessdata.fda.gov/scripts/cdrh/cfdocs/cfpma/pma.cfm?id=P130021S076
- Yoon SH, Kim WK, Dhoble A, et al. Bicuspid Aortic Valve Morphology and Outcomes After Transcatheter Aortic Valve Replacement. J Am Coll Cardiol 2020;76:1018-30. [Crossref] [PubMed]
- Boodhwani M, de Kerchove L, Glineur D, et al. Repair of regurgitant bicuspid aortic valves: a systematic approach. J Thorac Cardiovasc Surg 2010;140:276-284.e1. [Crossref] [PubMed]
- Beckerman Z, Kayatta MO, McPherson L, et al. Bicuspid aortic valve repair in the setting of severe aortic insufficiency. J Vis Surg 2018;4:101. [Crossref] [PubMed]
- Liu X, He Y, Zhu Q, et al. Supra-annular structure assessment for self-expanding transcatheter heart valve size selection in patients with bicuspid aortic valve. Catheter Cardiovasc Interv 2018;91:986-94. [Crossref] [PubMed]
- Kodali S, Pibarot P, Douglas PS, et al. Paravalvular regurgitation after transcatheter aortic valve replacement with the Edwards sapien valve in the PARTNER trial: characterizing patients and impact on outcomes. Eur Heart J 2015;36:449-56. [Crossref] [PubMed]
- Généreux P, Head SJ, Hahn R, et al. Paravalvular leak after transcatheter aortic valve replacement: the new Achilles' heel? A comprehensive review of the literature. J Am Coll Cardiol 2013;61:1125-36. [Crossref] [PubMed]
- Mauri V, Deuschl F, Frohn T, et al. Predictors of paravalvular regurgitation and permanent pacemaker implantation after TAVR with a next-generation self-expanding device. Clin Res Cardiol 2018;107:688-97. [Crossref] [PubMed]
- Khalique OK, Hahn RT, Gada H, et al. Quantity and location of aortic valve complex calcification predicts severity and location of paravalvular regurgitation and frequency of post-dilation after balloon-expandable transcatheter aortic valve replacement. JACC Cardiovasc Interv 2014;7:885-94. [Crossref] [PubMed]
- Daneault B, Koss E, Hahn RT, et al. Efficacy and safety of postdilatation to reduce paravalvular regurgitation during balloon-expandable transcatheter aortic valve replacement. Circ Cardiovasc Interv 2013;6:85-91. [Crossref] [PubMed]
- Fonseca P, Figueiredo B, Almeida C, et al. Aortic Valve Calcium Volume Predicts Paravalvular Regurgitation and the Need for Balloon Post-Dilatation After Transcatheter Aortic Valve Implantation. J Interv Cardiol 2016;29:117-23. [Crossref] [PubMed]
- Pasic M, Unbehaun A, Buz S, et al. Annular rupture during transcatheter aortic valve replacement: classification, pathophysiology, diagnostics, treatment approaches, and prevention. JACC Cardiovasc Interv 2015;8:1-9. [Crossref] [PubMed]
- Azadani AN, Chitsaz S, Matthews PB, et al. Comparison of mechanical properties of human ascending aorta and aortic sinuses. Ann Thorac Surg 2012;93:87-94. [Crossref] [PubMed]
- Leon MB, Mack MJ, Hahn RT, et al. Outcomes 2 Years After Transcatheter Aortic Valve Replacement in Patients at Low Surgical Risk. J Am Coll Cardiol 2021;77:1149-61. [Crossref] [PubMed]
- Mangione FM, Jatene T, Gonçalves A, et al. Leaflet Thrombosis in Surgically Explanted or Post-Mortem TAVR Valves. JACC Cardiovasc Imaging 2017;10:82-5. [Crossref] [PubMed]
- Yanagisawa R, Hayashida K, Yamada Y, et al. Incidence, Predictors, and Mid-Term Outcomes of Possible Leaflet Thrombosis After TAVR. JACC Cardiovasc Imaging 2016; [Epub ahead of print]. [PubMed]
- De Marchena E, Mesa J, Pomenti S, et al. Thrombus formation following transcatheter aortic valve replacement. JACC Cardiovasc Interv 2015;8:728-39. [Crossref] [PubMed]
- Makkar RR, Fontana G, Jilaihawi H, et al. Possible Subclinical Leaflet Thrombosis in Bioprosthetic Aortic Valves. N Engl J Med 2015;373:2015-24. [Crossref] [PubMed]
- Gunning PS, Vaughan TJ, McNamara LM. Simulation of self expanding transcatheter aortic valve in a realistic aortic root: implications of deployment geometry on leaflet deformation. Ann Biomed Eng 2014;42:1989-2001. [Crossref] [PubMed]
- Abbasi M, Azadani AN. Leaflet stress and strain distributions following incomplete transcatheter aortic valve expansion. J Biomech 2015;48:3663-71. [Crossref] [PubMed]
- Xuan Y, Dvir D, Wang Z, et al. Stent and leaflet stresses across generations of balloon-expandable transcatheter aortic valves. Interact Cardiovasc Thorac Surg 2020;30:879-86. [Crossref] [PubMed]
- Tchetche D, de Biase C, van Gils L, et al. Bicuspid Aortic Valve Anatomy and Relationship With Devices: The BAVARD Multicenter Registry. Circ Cardiovasc Interv 2019;12:e007107. [Crossref] [PubMed]
- Mangels DR, Siki M, Menon R, et al. Hemodynamic Effects of Valve Asymmetry in Sapien 3 Transcatheter Aortic Valves. J Invasive Cardiol 2018;30:138-43. [PubMed]
- Gunning PS, Saikrishnan N, McNamara LM, et al. An in vitro evaluation of the impact of eccentric deployment on transcatheter aortic valve hemodynamics. Ann Biomed Eng 2014;42:1195-206. [Crossref] [PubMed]
- Khodaee F, Barakat M, Abbasi M, et al. Incomplete expansion of transcatheter aortic valves is associated with propensity for valve thrombosis. Interact Cardiovasc Thorac Surg 2020;30:39-46. [Crossref] [PubMed]
- Hatoum H, Dollery J, Lilly SM, et al. Sinus Hemodynamics Variation with Tilted Transcatheter Aortic Valve Deployments. Ann Biomed Eng 2019;47:75-84. [Crossref] [PubMed]
- Chen S, Chau KH, Nazif TM. The incidence and impact of cardiac conduction disturbances after transcatheter aortic valve replacement. Ann Cardiothorac Surg 2020;9:452-67. [Crossref] [PubMed]
- Young Lee M, Chilakamarri Yeshwant S, Chava S, et al. Mechanisms of Heart Block after Transcatheter Aortic Valve Replacement - Cardiac Anatomy, Clinical Predictors and Mechanical Factors that Contribute to Permanent Pacemaker Implantation. Arrhythm Electrophysiol Rev 2015;4:81-5. [Crossref] [PubMed]
- Hamdan A, Guetta V, Klempfner R, et al. Inverse Relationship Between Membranous Septal Length and the Risk of Atrioventricular Block in Patients Undergoing Transcatheter Aortic Valve Implantation. JACC Cardiovasc Interv 2015;8:1218-28. [Crossref] [PubMed]
- Ribeiro HB, Webb JG, Makkar RR, et al. Predictive factors, management, and clinical outcomes of coronary obstruction following transcatheter aortic valve implantation: insights from a large multicenter registry. J Am Coll Cardiol 2013;62:1552-62. [Crossref] [PubMed]
- Khan JM, Greenbaum AB, Babaliaros VC, et al. The BASILICA Trial: Prospective Multicenter Investigation of Intentional Leaflet Laceration to Prevent TAVR Coronary Obstruction. JACC Cardiovasc Interv 2019;12:1240-52. [Crossref] [PubMed]
- Khan JM, Bruce CG, Babaliaros VC, et al. TAVR Roulette: Caution Regarding BASILICA Laceration for TAVR-in-TAVR. JACC Cardiovasc Interv 2020;13:787-9. [Crossref] [PubMed]
- Chen YH, Chang HH, Liao TW, et al. Membranous septum length predicts conduction disturbances following transcatheter aortic valve replacement. J Thorac Cardiovasc Surg 2022;164:42-51.e2. [Crossref] [PubMed]
- Snir A, Wilson MK, Ju LA, et al. Novel Pressure-Regulated Deployment Strategy for Improving the Safety and Efficacy of Balloon-Expandable Transcatheter Aortic Valves. JACC Cardiovasc Interv 2021;14:2503-15. [Crossref] [PubMed]
- Jilaihawi H, Zhao Z, Du R, et al. Minimizing Permanent Pacemaker Following Repositionable Self-Expanding Transcatheter Aortic Valve Replacement. JACC Cardiovasc Interv 2019;12:1796-807. [Crossref] [PubMed]
- Tang GHL, Zaid S, Michev I, et al. "Cusp-Overlap" View Simplifies Fluoroscopy-Guided Implantation of Self-Expanding Valve in Transcatheter Aortic Valve Replacement. JACC Cardiovasc Interv 2018;11:1663-5. [Crossref] [PubMed]
- Kohli K, Wei ZA, Yoganathan AP, et al. Transcatheter Mitral Valve Planning and the Neo-LVOT: Utilization of Virtual Simulation Models and 3D Printing. Curr Treat Options Cardiovasc Med 2018;20:99. [Crossref] [PubMed]
- Qian Z, Wang K, Liu S, et al. Quantitative Prediction of Paravalvular Leak in Transcatheter Aortic Valve Replacement Based on Tissue-Mimicking 3D Printing. JACC Cardiovasc Imaging 2017;10:719-31. [Crossref] [PubMed]
- Reiff C, Zhingre Sanchez JD, Mattison LM, et al. 3-Dimensional printing to predict paravalvular regurgitation after transcatheter aortic valve replacement. Catheter Cardiovasc Interv 2020;96:E703-10. [Crossref] [PubMed]
- Tzamtzis S, Viquerat J, Yap J, et al. Numerical analysis of the radial force produced by the Medtronic-CoreValve and Edwards-SAPIEN after transcatheter aortic valve implantation (TAVI). Med Eng Phys 2013;35:125-30. [Crossref] [PubMed]
- Bianchi M, Marom G, Ghosh RP, et al. Patient-specific simulation of transcatheter aortic valve replacement: impact of deployment options on paravalvular leakage. Biomech Model Mechanobiol 2019;18:435-51. [Crossref] [PubMed]
- Heitkemper M, Hatoum H, Azimian A, et al. Modeling risk of coronary obstruction during transcatheter aortic valve replacement. J Thorac Cardiovasc Surg 2020;159:829-838.e3. [Crossref] [PubMed]
- Dowling C, Bavo AM, El Faquir N, et al. Patient-Specific Computer Simulation of Transcatheter Aortic Valve Replacement in Bicuspid Aortic Valve Morphology. Circ Cardiovasc Imaging 2019;12:e009178. [Crossref] [PubMed]
- Lavon K, Marom G, Bianchi M, et al. Biomechanical modeling of transcatheter aortic valve replacement in a stenotic bicuspid aortic valve: deployments and paravalvular leakage. Med Biol Eng Comput 2019;57:2129-43. [Crossref] [PubMed]
- Mao W, Wang Q, Kodali S, et al. Numerical Parametric Study of Paravalvular Leak Following a Transcatheter Aortic Valve Deployment Into a Patient-Specific Aortic Root. J Biomech Eng 2018; [Crossref] [PubMed]
- Wu MCH, Muchowski HM, Johnson EL, et al. Immersogeometric fluid-structure interaction modeling and simulation of transcatheter aortic valve replacement. Comput Methods Appl Mech Eng 2019;357:112556. [Crossref] [PubMed]
- Lalys F, Esneault S, Castro M, et al. Automatic aortic root segmentation and anatomical landmarks detection for TAVI procedure planning. Minim Invasive Ther Allied Technol 2019;28:157-64. [Crossref] [PubMed]
- Galli V, Loncaric F, Rocatello G, et al. Towards patient-specific prediction of conduction abnormalities induced by transcatheter aortic valve implantation: a combined mechanistic modelling and machine learning approach. European Heart Journal-Digital Health 2021;2:606-15. [Crossref]
- Aggarwal A, Ferrari G, Joyce E, et al. Architectural trends in the human normal and bicuspid aortic valve leaflet and its relevance to valve disease. Ann Biomed Eng 2014;42:986-98. [Crossref] [PubMed]
- Emendi M, Sturla F, Ghosh RP, et al. Patient-Specific Bicuspid Aortic Valve Biomechanics: A Magnetic Resonance Imaging Integrated Fluid-Structure Interaction Approach. Ann Biomed Eng 2021;49:627-41. [Crossref] [PubMed]
- Luraghi G, Migliavacca F, García-González A, et al. On the Modeling of Patient-Specific Transcatheter Aortic Valve Replacement: A Fluid-Structure Interaction Approach. Cardiovasc Eng Technol 2019;10:437-55. [Crossref] [PubMed]
- Lee JH, Rygg AD, Kolahdouz EM, et al. Fluid-Structure Interaction Models of Bioprosthetic Heart Valve Dynamics in an Experimental Pulse Duplicator. Ann Biomed Eng 2020;48:1475-90. [Crossref] [PubMed]