Pathology and pathophysiology of the aortic root
Introduction
The aortic root spanning from the annulus to the sinotubular junction, described as the functional aortic annulus, is a complex structure that houses the aortic valve and coronary arteries. In pathological states (reviewed here), the wall of the aorta dilates, forming an aneurysm. The consequences of aneurysm formation in the root include aortic dissection, rupture, and aortic insufficiency (AI), and we will focus on each of these disease processes. Notably, these disease processes may be elegantly addressed by the aortic valve sparing root operation, the subject of this special issue. As such, aortic stenosis and endocarditis, which are also pathologies of the aortic root but not pertinent to the aortic valve sparing operation, will not be reviewed.
Normal structure and function of the aortic root
The aortic wall is comprised of multiple concentric layers, the innermost of which is the intima, followed by the media, and then the adventitia as the outermost layer. The intima is a single layer of endothelial cells that sits on the internal elastic lamina, which divides the intima from the media. The internal elastic lamina consists of type IV collagen and laminin. Important functions of the intima include serving as a permeable barrier and providing thromboresistance. It also mediates vascular tone through secretion of prostacyclin and endothelium-derived relaxing factor, while participating in inflammatory and immune responses (1). The adventitia largely consists of type 1 collagen and is often cited as the strength layer. It serves as the final barrier against rupture under extreme loading conditions.
The bulk of the aortic wall is the media, which is 80% of the wall and composed of collagen fibers, elastic fibers, smooth muscle cells and other molecules organized into concentric lamellar units. Concentric sheets of elastin sandwich smooth muscles cells and are connected by finer elastic fibers throughout (Figure 1). Collagen fibers are also interspersed and aligned circumferentially. These lamellar units are conserved across mammalian species and in normal aortas, relatively constant tension is carried per lamellar unit (2). The number of lamellar units is proportional to the radius of the aorta, and in humans there are roughly 50–70 such units in the thoracic aorta.
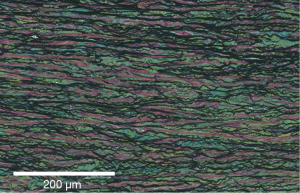
The aorta is therefore a complex compound material and its microstructure, especially that of the media, defines its biomechanical behaviour. The normal aorta, including the root, is able to withstand cyclic loading of 2.5 billion heartbeats in a lifetime. The normal aorta easily accommodates exercise and elevations in blood pressure. It is a compliant vessel that expands during systole and exhibits elastic recoil during diastole where the vessel reliably returns to its original configuration at the end of the cardiac cycle. Aortic stress-strain behaviour is non-linear, with the elastin networks defining the behaviour of the vessel at low strains. Collagen networks contribute to the steeper portion of stress-strain curve at high strains. Smooth muscle cells have well-defined roles in extracellular matrix (ECM) synthesis, secretion and turnover. These cells are linked to the ECM, forming elastin-contractile units that contract in response to pulsatile blood and are part of cell-signalling pathways such as the transforming growth factor-β (TGF-β) pathway (important in the formation of thoracic aortic disease) (3). Glycosaminoglycans also play a role, occupying 1% to 5% of the normal aortic wall. They are strongly negatively charged, thus sequestering water and contributing to the aorta’s ability to resist compressive radial forces. Pooling of glycosaminoglycans is seen in degeneration of the media and may play an important role in dissection initiation (4).
The histology and biomechanics vary along the length of the aorta with the more proximal segments of the aorta being more abundant in elastic fibers. For this reason, the proximal aorta is more compliant than the distal descending and abdominal aorta. However, the aortic root is not merely an extension of the ascending aorta. Histological comparison of 14 normal human aortic roots to the adjacent ascending aorta showed that both elastin and collagen components were more irregular in the aortic root, and that the sinuses of Valsalva were thinner than the ascending aorta by about half a millimeter (5). The same study found that the sinuses of Valsalva were stiffer than their ascending counterparts (5). Along the cusp insertion line, dense collagen fibers provide reinforcement and are continuous with the three thin fibrous interleaflet triangles that form the distal-most aspect of the left ventricular outflow tract. The entire root structure undergoes non-uniform multi-dimensional deformation throughout the cardiac cycle.
The shape of the sinuses of Valsalva facilitates closure of the aortic valve. The speed of blood closer to the aortic wall is slower and upon reaching the sinotubular junction, the blood swirls downwards towards the valve as first described by Leonardo Da Vinci. Hydrodynamic studies of the aortic root suggest that its unique shape and biomechanical properties minimize stress and deformation on the aortic cusps (6). This diastolic blood flow also supplies the myocardium through the coronary arteries.
Aneurysms of the aortic root
Aneurysms of the aortic root are often the result of heritable thoracic aortic disease (HTAD), which represent up to 20% of all thoracic aortic disease. HTAD may be either syndromic, such the prototypical Marfan syndrome with its multisystem involvement, or non-syndromic. They are caused by single gene mutations that are highly penetrant, behaving in an autosomal dominant manner. Although HTAD can affect the distal aorta, there is a proclivity for the aortic root. For example, in Marfan syndrome, which is most often caused by an FBN1 mutation, 81% of patients have dilated aortic roots (7). There can be sinotubular junction effacement and involvement of the ascending aorta, but rarely is it confined to the ascending aorta alone. Loeys-Dietz syndrome, which can be caused by mutations in several genes including TGFBR1 and TGFBR2, nearly universally presents with aortic root dilatation (8).
Due to pioneering work by Loeys, Dietz, Milewicz and others, in concert with advances in gene sequencing from Sanger sequencing to Next Gen sequencing, a significant number of genes have been attributed to HTAD. They may be grouped into (I) those affecting the ECM, (II) those affecting the TGF-β pathway, and (III) those affecting smooth muscle cell contraction (Table 1). The important TGF-β signalling pathway deserves special mention and is reviewed in detail elsewhere (9). TGF-β is a soluble peptide with a wide range of effects in multiple organ systems. It binds to type I and type II receptors. When the type II receptor is activated, it recruits a type I receptor, that in turn activates a receptor-Smad (r-Smad). The r-Smad forms a complex which translocates into the nucleus. This cascade then results in inducing and suppressing multiple genes involved in ECM deposition and degradation (Figure 2). Alternative Smad-independent pathways are described.
Table 1
Extracellular matrix |
FBN1 |
MFAPS |
LOX |
TGF-β pathway |
TGFBR1 |
TGFBR2 |
SMAD3 |
SMAD4 |
TGFB2 |
TGFB3 |
SMAD2 |
Smooth muscle cell contraction |
ACTA2 |
MYH11 |
MYLK |
PRKG1 |
MAT2A |
FOXE3 |
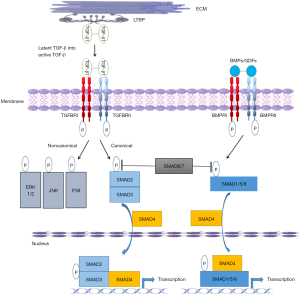
Tying this pathway back to Marfan syndrome and mutations in FBN1, fibrillin-1 forms microfibrils, which are not only important for their role in elastic fiber formation, but also in TGF-sequestration and therefore regulation of this cytokine. The observation that the renin-angiotensin system and angiotensin-II activation result in kidney fibrosis, in part due to complex interactions with the TGF-β pathway, sparked interest in understanding these interactions in the aorta. Habashi and colleagues found that losartan, an angiotensin receptor blocker that antagonizes TGF-β, prevented aortic aneurysm formation in a Marfan syndrome mouse model (10). This then led to a randomized controlled trial of patients with Marfan syndrome (COMPARE), where 233 patients were allocated to treatment with the angiotensin-II receptor blocker losartan in addition to beta-blockers or beta-blockers alone for 3 years (11). Those in the losartan group had lower blood pressures and experienced less aortic root enlargement. After a median follow-up of 8 years, in a smaller and more limited cohort, fewer of the composite aortic endpoints were reached in those who used losartan and this was driven by death and aortic dissection (12). Although there are important limitations to COMPARE, it nicely illustrates how understanding the pathology and pathophysiology of aortic root aneurysms can pave the way for real clinical impact.
Aortic root aneurysms may also be sporadic in that they do not behave in a hereditary pattern. The etiology for these aneurysms is degenerative or hypertension-related, but it is unclear why some degenerative aneurysms affect this segment of the aorta while others affect the ascending and beyond. For the diagnosis of sporadic aneurysms, it is important to recognize that there is a normal spectrum in aortic root dimensions and that they increase with age and patient size and are generally larger in men than women (13). Taking this into account, as a rule of thumb, it is exceedingly uncommon for aortic roots to be larger than 40 mm in women and 45 mm in men, and therefore aortic roots beyond these sizes should be treated as pathological.
With both aneurysmal degeneration and aging, the media of the aorta degenerates. The elastin sheets become fragmented, and there is loss of smooth muscle cell, collapsing the lamellar units (14). Pooling of proteoglycans and glycosaminoglycans is also observed. Increasing severity of the degree of medial degeneration has been correlated with alterations in the mechanical properties of the aortic wall, leaving it vulnerable to complications such as dissection and rupture (15-17). Hemodynamic forces applying shear stress to the aortic wall have also been linked to regional changes in medial degeneration and biomechanical properties (18,19).
Aortic insufficiency
AI can result from either geometric changes to the aorta or leaflet pathology, or a combination of the two. Understanding the pathology driving the AI is key to successful repair of the valve.
With respect to the aortic changes leading to AI, configurational changes at multiple levels can impact competence of the valve (Figure 3). At the annular level, dilation is termed annuloaortic ectasia and is seen in many conditions that result in HTAD, as described in the previous section. Finite element analysis models have demonstrated that an enlarged annulus decreases the effective heights of the aortic cusps, and reduces coaptation areas from 27% with an annulus of 20 mm, to 2.8% with an annulus of 30 mm (20).
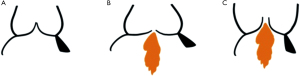
Distraction of the sinotubular junction is seen in both primary aneurysms of the aortic root, as well as aneurysms of the ascending aorta that secondarily extend into the aortic root. The resulting increased intercommissural distance leads to reduced cusp coaptation and AI. It is important to note that isolated changes to the sinuses of Valsalva without annular or sinotubular involvement do not result in AI.
Primary cusp pathology can result in AI. In a series of surgically explanted native valves, 14% of patients presented with pure AI: 44% were caused by non-inflammatory aortic root and/or cusp disease, 40% were related to infective endocarditis and 16% were related to rheumatic heart disease (21). Rheumatic valves are retracted while infective endocarditis is associated with cusp perforations and tears. Thus, it is this noninflammatory category of root and cusp disease that is most amenable to valve repair due to relative preservation of cusp tissue. These cusps demonstrate swelling of the spongiosa from myxomatous infiltration, fibrous lamina disruption, and free-edge fibrosis on histological examination (21). This results in redundant floppy cusps that prolapse into the left ventricular outflow tract during diastole. Cusp pathology may be more common than previously recognized. Cusp prolapse was found to be present in 81% of patients undergoing root surgery for aneurysm or AI with tricuspid aortic valves (22). It involved one cusp in 38% of cases, two cusps in 45% of cases and all cusps in 17% of cases. The most common prolapsed cusp was the right in 57% of cases.
Bicuspid aortic valves (BAVs) may cause AI and represent 20% of those presenting for surgery for pure AI (23). Calcific aortic stenosis is actually more common in BAV disease; among those with BAVs presenting for surgery, pure AI represented 13% (24). The Sievers classification describes the number of raphe that the patient has. Sievers 1 with a single raphe fusing two cusps together is the most common configuration, seen in 90% of patients (25). Elongation of the free margin of the fused cusp and prolapse of the fused cusp is the primary mechanism of aortic regurgitation in BAVs. However, concomitant prolapse of the non-fused cusp is also frequently present and may also require intervention at the time of repair (26). It is important to note that aortic dilation at the level of the annulus, root and ascending aorta, is commonly observed with BAVs regardless of valve function. In an echocardiography study, 59% of BAVs with severe AI were associated with dilation of the annulus, 78% with dilation of the sinuses and 79% with dilation of the sinotubular junction (27). Thus, multilevel intervention is commonly required in repair of BAVs with insufficiency.
The hemodynamic consequence of chronic AI is increased volume and pressure loading of the left ventricle. The regurgitant volume means that total stroke volume increases to maintain the same cardiac output. Increased total stroke volume results in systolic hypertension that exacerbates the left ventricular overload from the regurgitant volume itself. To adapt, the left ventricle undergoes eccentric hypertrophy where cardiac muscle mass increases while maintaining the volume/mass ratio.
Untreated, the prognosis of severe AI is poor. Longitudinal follow-up of patients with severe AI at the Mayo Clinic found that at 10 years, 75% of patients had died or had undergone aortic valve surgery, and 83% suffered cardiovascular events (28). This foundational observational research informed much of the current clinical valve guidelines with respect to timing of surgical intervention (29). The observations that development of heart failure symptoms, reduction in left ventricular ejection fraction <55%, and increased left ventricular dimensions (end-systolic diameter normalized to body surface area ≥25 mm/m2) were poor prognostic indicators prompted the currently used triggers for surgical intervention. More recent magnetic resonance imaging (MRI) data demonstrate divergence of long-term outcomes and dramatically worse survival for patients with regurgitant fractions >33% than those with less (30). MRI provides accurate left ventricular size data, and a drop in long term survival is also seen in those with left ventricular end diastolic volumes of >246 mL (30). It is clear that there is a precipice beyond which deleterious left ventricular remodeling is irreversible and impacts prognosis. Further refinement of how we identify when to intervene on individual patients is needed.
Aortic dissection
In patients who suffer acute aortic syndromes, a combination of increased aortic wall stress and structural weakening of the aortic wall results in failure of the aortic tissue. In the proximal aorta, this most frequently manifests as an aortic dissection. A primary intimal tear occurs allowing for blood to track into the aortic wall itself and delaminate the layers of the aortic wall along lamellae, creating a true and false lumen for blood flow. Many additional entry and re-entry tears may exist but there often is one primary tear that is larger than the others. Nevertheless, this dominant account of the pathophysiology of aortic dissection is challenged by alternate theories involving rupturing of vasa vasorum leading to accumulation of blood within the media which then secondarily extends through the intima (31). Another theory suggests that stress concentrations within the media are generated by the pooling of glycosaminoglycans/proteoglycans, a unique hallmark feature of medial degeneration of thoracic aortic aneurysms (4). These foci of stress then predispose the aorta to delamination. Given the impossibility of directly observing the initial seconds of an aortic dissection thus far, the exact pathophysiology will continue to be debated (although a combination of interrelated factors is more likely to be true than any single unifying theory).
The pathogenesis of the primary intimal tear is further complicated by the fact that its location frequently does not match areas of the largest dimensions. In other words, the tear often does not occur in the aortic root even for those who present with aortic root aneurysms. In fact, in a comprehensive computed tomography study, patients with primary intimal tears in the distal ascending aorta had the largest aortic roots (32). Even if a local area of vulnerability can be identified, what provokes an aortic dissection to occur when it happens is also unclear. In a retrospective series of telephone interviews with patients who had suffered acute aortic dissection, a third of patients were neither participating in anything strenuous nor experiencing any emotional stress (33).
Although the exact pathogenesis may still elude us, once an acute aortic dissection occurs, it is a medical emergency. When the ascending aorta (including the aortic root) is involved in the dissection, it is classified as a Stanford type A aortic dissection. The lethality of type A dissections was historically 1% per hour in the first 48 hours and remains high at 0.5% per hour in the first 48 hours according to the latest report from the International Registry of Aortic Dissection (IRAD) (34). Early surgery has a tremendous impact on reducing mortality and morbidity. Encouragingly, temporal analyses have shown higher rates of operative repair coupled with improved surgical outcomes over time (35-37). Negative predictors of outcome include shock, a comatose state and organ malperfusion (38).
While the need for timely surgery is universally accepted, diagnosis remains difficult in the emergency department due to the wide-ranging consequences of aortic wall delamination and variable presentation. These consequences include the potential for rupture, end-organ malperfusion and aortic valve insufficiency. Rupture from the acutely weakened and torn tissue can result in tamponade, syncope, and hemodynamic collapse. This is likely the mechanism of death for the approximately 50% of patients who die before reaching a hospital (39).
Malperfusion secondary to aortic dissection may involve any organ and therefore its presentation ranges from stroke to renal failure to acute limb ischemia. Malperfusion occurs through multiple mechanisms and is classically categorized as dynamic or static. In dynamic malperfusion, the inner partial thickness flap lifted by aortic wall delamination can be highly mobile and close off orifices of branch vessels especially when the false lumen becomes pressurized. By re-establishing the preference for true lumen flow with proximal aortic surgery and resection of the primary intimal tear, dynamic malperfusion can be expected to resolve. Given its time-sensitive nature, septal fenestration or thoracic endovascular aortic repair are alternative strategies in dealing with this type of malperfusion. The goal of de-pressurizing the false lumen remains the same. On the other hand, static malperfusion occurs when the dissection extends into the branch vessels and sometimes thrombosis can result. A thrombosed branch vessel will not re-open after re-establishing preference for true lumen flow. This type of malperfusion may require direct branch vessel intervention to resolve. Strategies include branch vessel stenting, resection and reconstruction of the affected vessel, bypass, or embolectomy.
Aortic valve insufficiency in the setting of aortic dissection is most commonly not a pre-existing condition, although it can be through mechanisms involving aortic root aneurysms and cusp pathology as described above. More common mechanisms include prolapse of a massive dissection flap of the ascending aorta into the root interfering with the orifice of the aortic valve. The dissection flap may also extend down into the aortic root. Extension into the sinuses alone does not cause AI, but if the posts of the commissures are affected, then they would prolapse, causing cusp prolapse and therefore AI. Therefore, resuspension of the commissures and resection of the prolapsing flap can correct many instances of AI in this setting.
Aortic root intervention is performed in 34–41% of patients with acute type A aortic dissection in multicentre series (37,40). Aortic root replacement, including aortic valve-sparing root replacement at the time of aortic dissection, is indicated when there is an aneurysm of the aortic root (>45 mm), intimal tears in the aortic root, or aortic root rupture. The corollary is that selection of operation in the setting of aortic dissection always depends on the clinical condition of the patient. Extensive dissection into the non-aneurysmal root may be reconstructed using a variety of methods, usually involving felt and glue, but root replacement is also an alternative. The choice of intervention in the dissected, non-aneurysmal root should be tailored to the patient and depends on surgeon preference.
Defining risk of aortic dissection in patients with aortic root aneurysms is challenging, and the level of evidence in terms of recommendations for surgery to prevent dissection in the most recent guidelines is low (41). While much progress has been made in genetics, HTAD is only present in a fraction of patients who dissect (5% have Marfan syndrome). Identification of aortic root aneurysms is helpful, but it is well-established that many patients who dissect have small aneurysms not at size threshold for surgery. A fuller understanding of the pathophysiology of this disease is still needed. Returning to the concept that aortic dissection is a culmination of wall stress exceeding the strength of the aortic tissue, peak wall stress experienced by the aorta may be estimated using finite element analysis. Patient-specific imaging, most frequently computed tomography, is used to create representative models, which are then subjected to different loads. A recent finite element analysis of 46 patients with Marfan syndrome found that peak stresses in the aortic root had no relationship to its maximal dimensions (42). The other side of the coin is understanding patient-specific weakening of the aortic wall that make it vulnerable to delamination. The strength of the aortic wall to resist delamination has been correlated to patient age, presence of hypertension and aortic valve phenotype (15).
Conclusions
Moreso than in the ascending aorta, aortic root aneurysms have an important genetic component to their etiology. Many genes have been implicated and they can be categorized as those affecting the ECM, the TGF-β pathway, or smooth muscle cell contraction. Aortic root pathology may induce AI through multiple mechanisms including annular dilatation, sinotubular dilatation and primary cusp pathology. Aortic root aneurysms are also at risk of aortic dissection and rupture, but the exact pathogenesis and risk stratification continue to be investigated.
Acknowledgments
Funding: None.
Footnote
Conflicts of Interest: The author has no conflicts of interest to declare.
Open Access Statement: This is an Open Access article distributed in accordance with the Creative Commons Attribution-NonCommercial-NoDerivs 4.0 International License (CC BY-NC-ND 4.0), which permits the non-commercial replication and distribution of the article with the strict proviso that no changes or edits are made and the original work is properly cited (including links to both the formal publication through the relevant DOI and the license). See: https://creativecommons.org/licenses/by-nc-nd/4.0/.
References
- Stary HC, Blankenhorn DH, Chandler AB, et al. A definition of the intima of human arteries and of its atherosclerosis-prone regions. A report from the Committee on Vascular Lesions of the Council on Arteriosclerosis, American Heart Association. Circulation 1992;85:391-405. [Crossref] [PubMed]
- Wolinsky H, Glagov S. A lamellar unit of aortic medial structure and function in mammals. Circ Res 1967;20:99-111. [Crossref] [PubMed]
- Milewicz DM, Trybus KM, Guo DC, et al. Altered Smooth Muscle Cell Force Generation as a Driver of Thoracic Aortic Aneurysms and Dissections. Arterioscler Thromb Vasc Biol 2017;37:26-34. [Crossref] [PubMed]
- Humphrey JD. Possible mechanical roles of glycosaminoglycans in thoracic aortic dissection and associations with dysregulated transforming growth factor-β. J Vasc Res 2013;50:1-10. [Crossref] [PubMed]
- Azadani AN, Chitsaz S, Matthews PB, et al. Comparison of mechanical properties of human ascending aorta and aortic sinuses. Ann Thorac Surg 2012;93:87-94. [Crossref] [PubMed]
- De Paulis R, Salica A, Pisani G, et al. Hemodynamics of the aortic valve and root: implications for surgery. Ann Cardiothorac Surg 2013;2:40-3. [PubMed]
- Wozniak-Mielczarek L, Sabiniewicz R, Drezek-Nojowicz M, et al. Differences in Cardiovascular Manifestation of Marfan Syndrome Between Children and Adults. Pediatr Cardiol 2019;40:393-403. [Crossref] [PubMed]
- Loeys BL, Chen J, Neptune ER, et al. A syndrome of altered cardiovascular, craniofacial, neurocognitive and skeletal development caused by mutations in TGFBR1 or TGFBR2. Nat Genet 2005;37:275-81. [Crossref] [PubMed]
- Jones JA, Spinale FG, Ikonomidis JS. Transforming growth factor-beta signaling in thoracic aortic aneurysm development: a paradox in pathogenesis. J Vasc Res 2009;46:119-37. [Crossref] [PubMed]
- Habashi JP, Judge DP, Holm TM, et al. Losartan, an AT1 antagonist, prevents aortic aneurysm in a mouse model of Marfan syndrome. Science 2006;312:117-21. [Crossref] [PubMed]
- Groenink M, den Hartog AW, Franken R, et al. Losartan reduces aortic dilatation rate in adults with Marfan syndrome: a randomized controlled trial. Eur Heart J 2013;34:3491-500. [Crossref] [PubMed]
- van Andel MM, Indrakusuma R, Jalalzadeh H, et al. Long-term clinical outcomes of losartan in patients with Marfan syndrome: follow-up of the multicentre randomized controlled COMPARE trial. Eur Heart J 2020;41:4181-7. [Crossref] [PubMed]
- Devereux RB, de Simone G, Arnett DK, et al. Normal limits in relation to age, body size and gender of two-dimensional echocardiographic aortic root dimensions in persons ≥15 years of age. Am J Cardiol 2012;110:1189-94. [Crossref] [PubMed]
- Halushka MK, Angelini A, Bartoloni G, et al. Consensus statement on surgical pathology of the aorta from the Society for Cardiovascular Pathology and the Association For European Cardiovascular Pathology: II. Noninflammatory degenerative diseases - nomenclature and diagnostic criteria. Cardiovasc Pathol 2016;25:247-57. [Crossref] [PubMed]
- Chung JC, Wong E, Tang M, et al. Biomechanics of Aortic Dissection: A Comparison of Aortas Associated With Bicuspid and Tricuspid Aortic Valves. J Am Heart Assoc 2020;9:e016715. [Crossref] [PubMed]
- Chung J, Lachapelle K, Wener E, et al. Energy loss, a novel biomechanical parameter, correlates with aortic aneurysm size and histopathologic findings. J Thorac Cardiovasc Surg 2014;148:1082-8; discussion 1088-9. [Crossref] [PubMed]
- Sherifova S, Holzapfel GA. Biomechanics of aortic wall failure with a focus on dissection and aneurysm: A review. Acta Biomater 2019;99:1-17. [Crossref] [PubMed]
- Salmasi MY, Pirola S, Sasidharan S, et al. High Wall Shear Stress can Predict Wall Degradation in Ascending Aortic Aneurysms: An Integrated Biomechanics Study. Front Bioeng Biotechnol 2021;9:750656. [Crossref] [PubMed]
- McClarty D, Ouzounian M, Tang M, et al. Ascending aortic aneurysm haemodynamics are associated with aortic wall biomechanical properties. Eur J Cardiothorac Surg 2022;61:367-75. [Crossref] [PubMed]
- Marom G, Haj-Ali R, Rosenfeld M, et al. Aortic root numeric model: annulus diameter prediction of effective height and coaptation in post-aortic valve repair. J Thorac Cardiovasc Surg 2013;145:406-411.e1. [Crossref] [PubMed]
- Agozzino L, de Vivo F, Falco A, et al. Surgical pathology of the aortic valve: gross and histological findings in 1120 excised valves. Cardiovasc Pathol 1994;3:155-61. [Crossref] [PubMed]
- Ehrlich T, Hagendorff A, Abeln K, et al. Aortic cusp abnormalities in patients with trileaflet aortic valve and root aneurysm. Heart 2022;109:55-62. [Crossref] [PubMed]
- Olson LJ, Subramanian R, Edwards WD. Surgical pathology of pure aortic insufficiency: a study of 225 cases. Mayo Clin Proc 1984;59:835-41. [Crossref] [PubMed]
- Sabet HY, Edwards WD, Tazelaar HD, et al. Congenitally bicuspid aortic valves: a surgical pathology study of 542 cases (1991 through 1996) and a literature review of 2,715 additional cases. Mayo Clin Proc 1999;74:14-26. [Crossref] [PubMed]
- Sillesen AS, Vøgg O, Pihl C, et al. Prevalence of Bicuspid Aortic Valve and Associated Aortopathy in Newborns in Copenhagen, Denmark. JAMA 2021;325:561-7. [Crossref] [PubMed]
- Aicher D, Kunihara T, Abou Issa O, et al. Valve configuration determines long-term results after repair of the bicuspid aortic valve. Circulation 2011;123:178-85. [Crossref] [PubMed]
- Hahn RT, Roman MJ, Mogtader AH, et al. Association of aortic dilation with regurgitant, stenotic and functionally normal bicuspid aortic valves. J Am Coll Cardiol 1992;19:283-8. [Crossref] [PubMed]
- Dujardin KS, Enriquez-Sarano M, Schaff HV, et al. Mortality and morbidity of aortic regurgitation in clinical practice. A long-term follow-up study. Circulation 1999;99:1851-7. [Crossref] [PubMed]
- Otto CM, Nishimura RA, Bonow RO, et al. 2020 ACC/AHA Guideline for the Management of Patients With Valvular Heart Disease: A Report of the American College of Cardiology/American Heart Association Joint Committee on Clinical Practice Guidelines. Circulation 2021;143:e72-e227. [PubMed]
- Myerson SG, d'Arcy J, Mohiaddin R, et al. Aortic regurgitation quantification using cardiovascular magnetic resonance: association with clinical outcome. Circulation 2012;126:1452-60. [Crossref] [PubMed]
- Haverich A, Boyle EC. Aortic dissection is a disease of the vasa vasorum. JTCVS Open 2021;5:30-2. [Crossref] [PubMed]
- Takami Y, Tajima K, Kato W, et al. Can we predict the site of entry tear by computed tomography in patients with acute type a aortic dissection? Clin Cardiol 2012;35:500-4. [Crossref] [PubMed]
- Hatzaras IS, Bible JE, Koullias GJ, et al. Role of exertion or emotion as inciting events for acute aortic dissection. Am J Cardiol 2007;100:1470-2. [Crossref] [PubMed]
- Harris KM, Nienaber CA, Peterson MD, et al. Early Mortality in Type A Acute Aortic Dissection: Insights From the International Registry of Acute Aortic Dissection. JAMA Cardiol 2022;7:1009-15. [Crossref] [PubMed]
- Benedetto U, Sinha S, Dimagli A, et al. Decade-long trends in surgery for acute Type A aortic dissection in England: A retrospective cohort study. Lancet Reg Health Eur 2021;7:100131. [Crossref] [PubMed]
- Ibrahim M, Stevens LM, Ouzounian M, et al. Evolving Surgical Techniques and Improving Outcomes for Aortic Arch Surgery in Canada. CJC Open 2021;3:1117-24. [Crossref] [PubMed]
- Evangelista A, Isselbacher EM, Bossone E, et al. Insights From the International Registry of Acute Aortic Dissection: A 20-Year Experience of Collaborative Clinical Research. Circulation 2018;137:1846-60. [Crossref] [PubMed]
- Conzelmann LO, Weigang E, Mehlhorn U, et al. Mortality in patients with acute aortic dissection type A: analysis of pre- and intraoperative risk factors from the German Registry for Acute Aortic Dissection Type A (GERAADA). Eur J Cardiothorac Surg 2016;49:e44-52. [Crossref] [PubMed]
- Mahase E. Half of patients with acute aortic dissection in England die before reaching a specialist centre. BMJ 2020;368:m304. [Crossref] [PubMed]
- Hage F, Hage A, Dagenais F, et al. Does adding an aortic root replacement or sinus repair during arch repair increase postoperative mortality? Evidence from the Canadian Thoracic Aortic Collaborative. Eur J Cardiothorac Surg 2021;60:623-30. [Crossref] [PubMed]
- Isselbacher EM, Preventza O, Hamilton Black J 3rd, et al. 2022 ACC/AHA Guideline for the Diagnosis and Management of Aortic Disease: A Report of the American Heart Association/American College of Cardiology Joint Committee on Clinical Practice Guidelines. Circulation 2022;146:e334-482. [Crossref] [PubMed]
- Xuan Y, D'Souza SN, Wang Z, et al. Patient-Specific Biomechanics in Marfan Ascending Thoracic Aortic Aneurysms. Ann Thorac Surg 2022;114:1367-75. [Crossref] [PubMed]